Advanced information
Scientific Background:
Machinery Regulating Vesicle Traffic, A Major Transport System in our Cells (pdf)

Scientific Background
Machinery Regulating Vesicle Traffic, A Major Transport System in our Cells
The 2013 Nobel Prize in Physiology or Medicine is awarded to Dr. James E. Rothman, Dr. Randy W. Schekman and Dr. Thomas C. Südhof for their discoveries of machinery regulating vesicle traffic, a major transport system in our cells. This represents a paradigm shift in our understanding of how the eukaryotic cell, with its complex internal compartmentalization, organizes the routing of molecules packaged in vesicles to various intracellular destinations, as well as to the outside of the cell. Specificity in the delivery of molecular cargo is essential for cell function and survival. This specificity is required for the release of neurotransmitters into the presynaptic region of a nerve cell to transmit a signal to a neighboring nerve cell. Likewise, specificity is required for the export of hormones such as insulin to the cell surface. While vesicles within the cell were long known to be critical components of this transportation scheme, the precise mechanism by which these vesicles found their correct destination and how they fused with organelles or the plasma membrane to deliver the cargo remained mysterious. The work of the three 2013 Laureates radically altered our understanding of this aspect of cell physiology. Randy W. Schekman used yeast genetics to identify a set of genes critical for vesicular trafficking. He showed that these genes were essential for life and could be classified into three categories regulating different aspects of vesicle transport. James E. Rothman embarked on a biochemical approach and identified proteins that form a functional complex controlling cell fusion. Proteins on the vesicle and target membrane sides bind in specific combinations, ensuring precise delivery of molecular cargo to the right destination. Thomas C. Südhof became interested in how vesicle fusion machinery was controlled. He unraveled the mechanism by which calcium ions trigger release of neurotransmitters, and identified key regulatory components in the vesicle fusion machinery. Together, Rothman, Schekman and Südhof have transformed the way we view transport of molecular cargo to specific destinations inside and outside the cell. Their discoveries explain a long-standing enigma in cell biology and also shed new light on how disturbances in this machinery can have deleterious effects and contribute to conditions such as neurological diseases, diabetes, and immunological disorders.
Introduction
Eukaryotic cells differ from prokaryotic cells by their more complex intracellular organization. In eukaryotes, specific cellular functions are compartmentalized into the cell nucleus and organelles surrounded by intracellular membranes. This compartmentalization vastly improves the efficiency of many cellular functions and prevents potentially dangerous molecules from roaming freely within the cell. But when distinct cellular processes are compartmentalized, a problem emerges. Different compartments need to exchange specific molecules (Figure 1). Furthermore, certain molecules need to be exported to the cell exterior. Most molecules are too large to directly pass through membranes, thus a mechanism that ensures specific delivery of this molecular cargo is required.
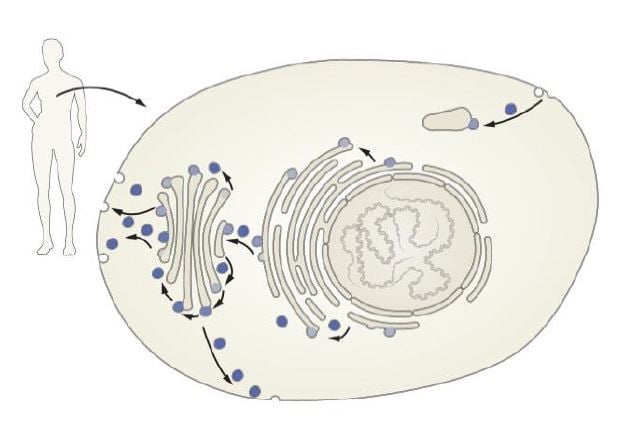
Mysteries of cellular compartmentalization have long intrigued scientists. Improved light microscopy techniques aided in the understanding of intracellular organization in eukaryotic cells, but the advent of electron microscopy and new staining techniques, combined with subcellular fractionation assays using differential ultracentrifugation procedures, led to a deeper understanding of the cell’s inner life. Albert Claude, George Palade and Christian de Duve, who received the Nobel Prize in Physiology or Medicine 1974*, were pioneers in this area and have shed light on how the cell is organized and compartmentalized. Secretory proteins were shown to be produced on ribosomes in the endoplasmic reticulum (ER) and trafficked to the Golgi complex (named after the 1906 Nobel Laureate Camillo Golgi) (Figure 1). Progress was also made in deciphering how proteins find their appropriate destination. Günter Blobel was awarded the 1999 Nobel Prize in Physiology or Medicine* for his discoveries that proteins have intrinsic signals that govern their transport and localization in the cell. Yet, a lingering question remained. How are molecules, including hormones, transport proteins, and neurotransmitters, correctly routed to their appropriate destination? From the work of Palade, the traffic of secretory proteins from the ER was understood to be carried out using small membrane-surrounded vesicles that bud from one membrane and fuse with another, but how precision could be acquired in this process remained enigmatic.
Identification of genes for vesicle fusion using yeast genetics
Randy W. Schekman, who trained in biochemistry with Arthur Kornberg (Nobel Prize 1959) decided to use yeast genetics, rather than biochemistry to dissect the mechanism involved in membrane and vesicle trafficking. Schekman realized that baker’s yeast (Saccharomyces cerevisiae) secretes glycoproteins and that this genetically amenable organism could therefore be used to study vesicle transport and fusion. Schekman devised a genetic screen to identify genes regulating intracellular transport. He reasoned that some of the mutations may be lethal, and to bypass the lethality problem, he used temperature-sensitive mutants and screened for genes affecting the intracellular accumulation of secretory enzymes (1-3).
In an initial version of the screen, Schekman identified two genes, sec1 and sec2, but when refined, the screen led to a further identification of 23 genes (2). Importantly the 23 genes could be assigned to three different classes, based on the accumulation of membranes that reflected blocks in traffic from the ER, the Golgi complex or in the specific case of sec1, to the cell surface (2) (Figure 2). The sequence of posttranslational events in the export of yeast glycoproteins was then determined with the aid of mutants that affect the secretory apparatus (3). Through a subsequent genetic and morphologic study of these mutants, Schekman discovered vesicle intermediates in the traffic between the ER and Golgi (4). Importantly, the sec17 and sec18 mutants accumulated small vesicles implicating a role in vesicle fusion.
Schekman provided a genetic basic for vesicle traffic and fusion by identifying key regulatory genes for vesicle traffic. He systematically unraveled the events along secretory pathways involved in vesicle traffic and in the interaction of trafficking vesicles with target membranes (Figure 2).
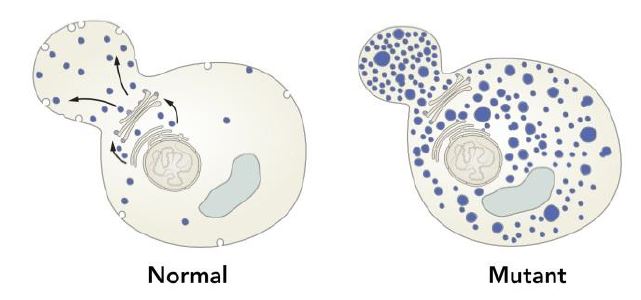
A biochemical journey to identify key proteins in the fusion process
James E. Rothman embarked upon a novel approach as a young group leader at Stanford University where he developed an in vitro reconstitution assay to dissect events involved in intracellular vesicle transport. Using this approach, he purified essential components of the vesicle fusion process. Given that in the 1970s it was difficult to express genes in animal cells, Rothman took advantage of a system based on vesicular stomatitis virus (VSV) that he had learned in Harvey Lodish´s laboratory at the Massachusetts Institute of Technology. In this system, large amounts of a particular viral protein, the VSV-G protein, are produced in infected cells. A unique feature of this system is that the VSV-G protein is marked by a particular sugar modification when it reaches the Golgi compartment, which makes it possible to identify when it reaches its destination. Rothman published a series of papers where he reconstituted the intracellular transport of the VSV-G protein within the Golgi complex (5-8). He then used the assay to study both vesicle budding and fusion, and purified proteins from the cytoplasm that were required for transport. The first protein to be purified was the N- ethylmaleimide-sensitive factor (NSF) (9-11).
Rothman’s discovery of NSF paved the way for the subsequent identification of other proteins important for the control of vesicle fusion, and the next one in line was SNAP (soluble NSF- attachment protein) (12). SNAPs bind to membranes and assist in the recruitment of NSF. An important point of convergence between Schekman’s and Rothman’s work was the discovery that one of the yeast mutants, sec18, corresponded to NSF (13, 14), which also revealed that the vesicle fusion machinery was evolutionarily ancient. Furthermore, Rothman and Schekman collaboratively cloned sec17 and provided evidence of its functional equivalence to SNAP (15). Other sec genes were shown to correspond to genes encoding fusion proteins were identified by other methods.
Using the NSF and SNAP proteins as bait, Rothman next turned to brain tissue, from which he purified proteins that he later named SNAREs (soluble NSF-attachment protein receptors) (16). Intriguingly, three SNARE proteins, VAMP/Synaptobrevin, SNAP-25 and syntaxin, were found in stoichiometric amounts, which suggested to Rothman that they functioned together in the vesicle and target membranes. The three proteins had previously been identified by several scientists, including Richard Scheller, Kimio Akagawa, Reinhard Jahn and Pietro de Camilli, and localized to the presynaptic region, but their function was largely unknown. VAMP/Synaptobrevin resided on the vesicle, whereas SNAP-25 and syntaxin were found at the plasma membrane. This prompted Rothman to propose a hypothesis – the SNARE hypothesis – which stipulated that target and vesicle SNAREs (t-SNAREs and v-SNAREs) were critical for vesicle fusion through a set of sequential steps of synaptic docking, activation and fusion (16). To test the SNARE hypothesis, Rothman used an in vitro reconstitution assay and revealed that SNAREs could indeed fuse membranes (17). He provided evidence that the system has a high degree of specificity, such that a particular t-SNARE only interacted with one or a few of the large number of potential v-SNAREs (18) (Figure 3). The SNARE hypothesis was critical to ignite the research field and the essence of the hypothesis, with its emphasis on the interaction between v-and t-SNAREs, has stood the test of time, although it has been mechanistically refined by several research groups.
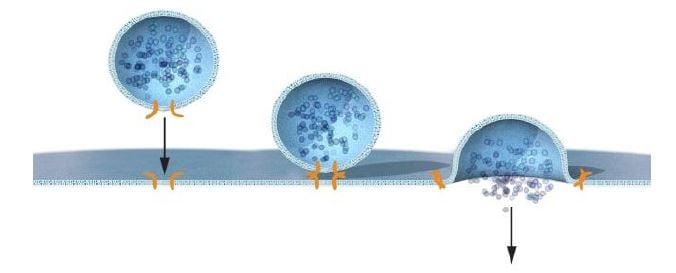
Rothman dissected the mechanism for vesicle transport and membrane fusion, and through biochemical studies proposed a model to explain how vesicle fusion occurs with the required specificity (Figure 3).
Identification of genes controlling the timing of vesicle fusion
Thomas C. Südhof originally trained at the Georg-August-Universität and the Max-Planck Institute for Biophysical Sciences in Göttingen, Germany, and was a postdoctoral fellow with Michael Brown and Joseph Goldstein (Nobel Prize 1985) at University of Texas Southwestern Medical School in Dallas. As a junior group leader, he set out to study how synaptic vesicle fusion was controlled. Rothman and Schekman had provided fundamental machinery for vesicle fusion, but how vesicle fusion was temporally controlled remained enigmatic. Vesicular fusions in the body need to be kept carefully in check, and in some cases vesicle fusion has to be executed with high precision in response to specific stimuli. This is the case for example for neurotransmitter release in the brain and for insulin secretion from the endocrine pancreas.
The neurophysiology field was electrified by the discoveries of Bernard Katz, Ulf von Euler and Julius Axelrod who received the Nobel Prize in Physiology or Medicine 1970* for their discoveries concerning the humoral transmittors in the nerve terminals and the mechanism for their storage, release and inactivation. Südhof was intrigued by the rapid exocytosis of synaptic vesicles, which is under tight temporal control and regulated by the changes in the cytoplasmic free calcium concentration. Südhof elucidated how calcium regulates neurotransmitter release in neurons and discovered that complexin and synaptotagmin are two critical proteins in calcium-mediated vesicle fusion (Figure 4).
Complexin competes with alpha-SNAP, but not synaptotagmin, for SNAP receptor binding (19). Neurons from complexin knock-out mice showed dramatically reduced transmitter release efficiency due to decreased calcium sensitivity of the synaptic secretion process (20). This revealed that complexin acts at a late step in synaptic fusion as a clamping mechanism that prevents constitutive fusion and allows regulated exocytosis to occur (20). Südhof also discovered synaptotagmin-1 (21), which coupled calcium to neurotransmitter release (22). Synaptotagmin-1 interacts with phospholipids in a calcium-dependent manner, as well as with syntaxin-1 and SNAREs, and Südhof established a role for synaptotagmin-1 as a calcium sensor for rapid synaptic fusion by elegantly demonstrating that calcium binding to synaptotagmin-1 participates in triggering neurotransmitter release at the synapse (23). Südhof also characterized Munc18-1, which corresponds to Schekman´s sec-1 and is therefore also called an SM protein (for Sec/Munc). Munc18-1 was found to interact with syntaxin (24) and later also to clasp the Trans-SNARE complex. SM proteins are now known to be an integral part of the vesicle fusion protein complex, along with the SNARE proteins. Südhof showed that deletion of Munc18-1 in mice leads to a complete loss of neurotransmitter secretion from synaptic vesicles (25).

Südhof made critical discoveries that advanced the understanding of how vesicle fusion is temporally controlled and he elucidated the ways that calcium levels regulate neurotransmitter release at the synapse (Figure 4).
Vesicle fusion and its importance for medicine
The work of Rothman, Schekman and Südhof has unraveled machinery that is essential for routing of cargo in cells in organisms as distantly related as yeast and man. These discoveries have had a major impact on our understanding of how molecules are correctly sorted to precise locations in cell. In the light of this, it comes as no surprise that defects at any number of steps in the machinery controlling vesicle transport and fusion are associated with disease.
Vesicle transport and fusion is essential for physiological processes ranging from control of nerve cell communication in the brain to immunological responses and hormone section. Deregulation of the transport system is associated with disease in these areas. For example, metabolic disorders such as type 2 diabetes are characterized by defects in both insulin secretion from pancreatic beta-cells and insulin-mediated glucose transporter translocation in skeletal muscle and adipose tissue. Furthermore, immune cells in our bodies rely on functional vesicle trafficking and fusion to send out substances including cytokines and immunologic effector molecules that mediate innate and adaptive immune responses.
In addition to these more general links between vesicle fusion and disease, specific mutations in the genes encoding the proteins in the vesicle fusion machinery give rise to a number of diseases. For example, in certain forms of epilepsy, mutations in the gene encoding MUNC-18-1 have been described. Likewise, in a subset of patients suffering from Familial Hemophagocytic Lymphohistiocytosis (FHL), mutations in the MUNC13-4, MUCH18-2 and syntaxin-11 genes have been found. In FHL patients, natural killer cells fail to regulate properly when encountering target cells, leading to hyperinflammation, which can be lethal. Furthermore, certain bacterial toxins target the vesicle fusion machinery. Botulism, caused by the anaerobic bacterium Clostridium botulinum, is a paralytic disease and the majority of the toxin types cleave SNAP-25, VAMP/Synaptobrevin and Syntaxin, thereby inhibiting acetylcholine release at the neuromuscular junction (26). The tetanus neurotoxin (from Clostridium tetani) targets VAMP/Synaptobrevin in inhibitory interneurons and blocks release of GABA or glycine, leading to spastic paralysis (26). Thus, the discoveries of Rothman, Schekman and Südhof have provided clarity into disease mechanisms and prospective treatments.
Conclusions
The discoveries by James E. Rothman, Randy W. Schekman and Thomas C. Südhof have elucidated some of the most fundamental processes in eukaryotic cells that collectively ensure that molecular cargo is correctly destined. Their discoveries have had a major impact on our understanding of how cellular communication occurs to sort molecules to precise locations within and outside the cell. Vesicle transport and fusion operates, with the same general principles, in organisms as different as yeast and man. It is critical for a variety of physiological processes in which vesicle fusion must be controlled, ranging from hormone or neurotransmitter release to functions of the immune system. Without this exquisitely precise organization, the cell would lapse into chaos.
Juleen R. Zierath and Urban Lendahl
Karolinska Institutet
Juleen R. Zierath
Professor of Clinical Integrative Physiology, Karolinska Institutet
Chairman of the Nobel Committee
Member of the Nobel Assembly
Urban Lendahl
Professor of Genetics, Karolinska Institutet
Adjunct Member of the Nobel Committee Member of the Nobel Assembly
Illustrations: Mattias Karlén
* Footnotes
The Nobel Prize in Physiology or Medicine 1970 to Bernard Katz, Ulf von Euler and Julius Axelrod “for their discoveries concerning the humoral transmittors in the nerve terminals and the mechanism for their storage, release and inactivation“
The Nobel Prize in Physiology or Medicine 1974 to Albert Claude, Christian de Duve and George E. Palade “for their discoveries concerning the structural and functional organization of the cell“
The Nobel Prize in Physiology or Medicine 1999 to Günter Blobel “for the discovery that proteins have intrinsic signals that govern their transport and localization in the cell“
References
- Novick P, Schekman R: Secretion and cell-surface growth are blocked in a temperature-sensitive mutant of Saccharomyces cerevisiae. Proc Natl Acad Sci USA 76:1858-1862, 1979
- Novick P, Field C, Schekman R: Identification of 23 complementation groups required for post-translational events in the yeast secretory pathway. Cell 21:205-215, 1980
- Novick P, Ferro S, Schekman R: Order of events in the yeast secretory pathway. Cell 25:461-469, 1981
- Kaiser CA, Schekman R: Distinct sets of SEC genes govern transport vesicle formation and fusion early in the secretory pathway. Cell 61:723-733, 1990
- Balch WE, Dunphy WG, Braell WA, Rothman JE: Reconstitution of the transport of protein between successive compartments of the Golgi measured by the coupled incorporation of N-acetylglucosamine. Cell 39:405-416, 1984
- Balch WE, Glick BS, Rothman JE: Sequential intermediates in the pathway of intercompartmental transport in a cell-free system. Cell 39:525-536, 1984
- Braell WA, Balch WE, Dobbertin DC, Rothman JE: The glycoprotein that is transported between successive compartments of the Golgi in a cell-free system resides in stacks of cisternae. Cell 39:511-524, 1984
- Fries E, Rothman JE: Transport of vesicular stomatitis virus glycoprotein in a cell-free extract. Proc Natl Acad Sci USA 77:3870-3874, 1980
- Block MR, Glick BS, Wilcox CA, Wieland FT, Rothman JE: Purification of an N-ethylmaleimide-sensitive protein catalyzing vesicular transport. Proc Natl Acad Sci USA 85:7852-7856, 1988
- Malhotra V, Orci L, Glick BS, Block MR, Rothman JE: Role of an N-ethylmaleimide-sensitive transport component in promoting fusion of transport vesicles with cisternae of the Golgi stack. Cell 54:221-227, 1988
- Glick BS, Rothman JE: Possible role for fatty acyl-coenzyme A in intracellular protein transport.
Nature 326:309-312, 1987 - Clary DO, Griff IC, Rothman JE: SNAPs, a family of NSF attachment proteins involved in intracellular membrane fusion in animals and yeast. Cell 61:709-721, 1990
- Wilson DW, Wilcox CA, Flynn GC, Chen E, Kuang WJ, Henzel WJ, Block MR, Ullrich A, Rothman JE: A fusion protein required for vesicle-mediated transport in both mammalian cells and yeast. Nature 339:355-359, 1989
- Eakle KA, Bernstein M, Emr SD: Characterization of a component of the yeast secretion machinery: identification of the SEC18 gene product. Mol Cell Biol 8:4098-4109, 1988
- Griff IC, Schekman R, Rothman JE, Kaiser CA: The yeast SEC17 gene product is functionally equivalent to mammalian alpha-SNAP protein. J Biol Chem 267:12106-12115, 1992
- Sollner T, Whiteheart SW, Brunner M, Erdjument-Bromage H, Geromanos S, Tempst P, Rothman JE: SNAP receptors implicated in vesicle targeting and fusion. Nature 362:318-324, 1993
- Weber T, Zemelman BV, McNew JA, Westermann B, Gmachl M, Parlati F, Sollner TH, Rothman JE: SNAREpins: minimal machinery for membrane fusion. Cell 92:759-772, 1998
- McNew JA, Parlati F, Fukuda R, Johnston RJ, Paz K, Paumet F, Sollner TH, Rothman JE: Compartmental specificity of cellular membrane fusion encoded in SNARE proteins. Nature 407:153-159, 2000
- McMahon HT, Missler M, Li C, Sudhof TC: Complexins: cytosolic proteins that regulate SNAP receptor function. Cell 83:111-119, 1995
- Reim K, Mansour M, Varoqueaux F, McMahon HT, Sudhof TC, Brose N, Rosenmund C: Complexins regulate a late step in Ca2+-dependent neurotransmitter release. Cell 104:71-81, 2001
- Perin MS, Fried VA, Mignery GA, Jahn R, Sudhof TC: Phospholipid binding by a synaptic vesicle protein homologous to the regulatory region of protein kinase C. Nature 345:260-263, 1990
- Geppert M, Goda Y, Hammer RE, Li C, Rosahl TW, Stevens CF, Sudhof TC: Synaptotagmin I: a major Ca2+ sensor for transmitter release at a central synapse. Cell 79:717-727, 1994
- Fernandez-Chacon R, Konigstorfer A, Gerber SH, Garcia J, Matos MF, Stevens CF, Brose N, Rizo J, Rosenmund C, Sudhof TC: Synaptotagmin I functions as a calcium regulator of release probability. Nature 410:41-49, 2001
- Hata Y, Slaughter CA, Sudhof TC: Synaptic vesicle fusion complex contains unc-18 homologue bound to syntaxin. Nature 366:347-351, 1993
- Verhage M, Maia AS, Plomp JJ, Brussaard AB, Heeroma JH, Vermeer H, Toonen RF, Hammer RE, van den Berg TK, Missler M, Geuze HJ, Sudhof TC: Synaptic assembly of the brain in the absence of neurotransmitter secretion. Science 287:864-869, 2000
- Schiavo G, Benfenati F, Poulain B, Rossetto O, Polverino de Laureto P, DasGupta BR, Montecucco C: Tetanus and botulinum-B neurotoxins block neurotransmitter release by proteolytic cleavage of synaptobrevin. Nature 359:832-835, 1992
Nobel Prizes and laureates
Six prizes were awarded for achievements that have conferred the greatest benefit to humankind. The 12 laureates' work and discoveries range from proteins' structures and machine learning to fighting for a world free of nuclear weapons.
See them all presented here.