Advanced information
Scientific background:
Avermectin and artemisinin – Revolutionary therapies against parasitic diseases (pdf)

Scientific Background
Avermectin and Artemisinin – Revolutionary Therapies against Parasitic Diseases
The 2015 Nobel Prize in Physiology or Medicine is awarded with one half jointly to Dr. William C. Campbell and Professor Satoshi Ōmura for their discoveries concerning a novel therapy against infections caused by roundworm parasites and the other half to Professor Tu Youyou for her discoveries concerning a novel therapy against Malaria. Parasitic diseases have long plagued humankind and constitute a major global health problem. River Blindness (Onchocerciasis) is a disease that can ultimately lead to irreversible blindness. Lymphatic Filariasis causes chronic swelling and leads to life-long stigmatizing and disabling clinical symptoms, known as Elephantiasis (Lymphedema) and Scrotal Hydrocele. Both these diseases are caused by parasitic worms. Malaria, a mosquito-borne disease, is caused by single-cell parasites (Plasmodium), which invade red blood cells, causing fever, and in severe cases brain inflammation and death. Collectively, these diseases affect the world’s most vulnerable citizens, particularly in sub-Saharan Africa, South Asia and South and Central America, and represent a huge barrier towards improving human health and welfare. The 2015 Nobel Laureates have radically altered the treatment of these devastating diseases. Satoshi Ōmura searched for bioactive substances from the soil and isolated a new microorganism (Streptomyces avermitilis) with remarkable properties. William C. Campbell identified the antiparasitic activity of Ōmura’s microbial culture and characterized the effective component, named Avermectin, against a variety of parasitic worms in domestic and farm animals. Today the Avermectin-derivative Ivermectin is on good way to eradicate River Blindness and Lymphatic Filariasis. Tu Youyou turned to traditional herbal medicine and ancient literature in her quest for a therapy against Malaria. She identified an extract from the plant Artemisia annua that inhibited parasite growth and isolated the active component (Artemisinin) that was highly effective against malaria. Today, Artemisinin-based drug combination therapy has profoundly reduced the incidence and mortality of malaria, saving millions of lives worldwide. Campbell, Ōmura and Tu have transformed the treatment of parasitic diseases. The global impact of their discoveries and benefit to mankind is immeasurable.
Microbes and Parasites – A Major Health Problem
A plethora of organisms, both of bacterial and parasitic origin, are harmful or deadly to us. The medical community has long searched for novel therapeutic approaches to combat bacterial infections. Gerhard Domagk discovered sulfonamides (Nobel Prize 1939*); Alexander Fleming discovered penicillin and Ernst Chain and Howard Flory isolated the active component, and showed it was effective in combating bacterial infections (Nobel Prize 1945*). Selman Waksman’s isolation of Streptomyces bacteria from soil led to his discovery of streptomycin against tuberculosis (Nobel Prize 1952*).
In contrast, for a long time, there was limited progress in developing well-functioning therapies for parasitic diseases. For a number of diseases, such as River Blindness and Lymphatic Filariasis there were no safe and effective therapies, and for several other diseases, including malaria, durability and resistance to conventional drugs remained an issue that hampered efforts to achieve long-lasting treatment. While there were global attempts to develop therapies, they were either not very effective or associated with side effects. This situation was radically changed by the discoveries made by the 2015 Nobel Laureates.
Parasitic Diseases and Impact on Global Health
Parasites are organisms that reside in or on another organism (their host), where they feed on nutrients, propagate, and often cause chronic or life-threatening diseases. There are three main classes of parasites that can cause disease in humans: protozoa, helminths, and ectoparasites. Parasitic diseases have plagued humans for as long as we know and constitute a major global health problem. In particular, parasitic diseases affect the world’s most vulnerable citizens and represent an enormous barrier to improving health and wellbeing (Figure 1). Many parasites also attack domestic animals and livestock, which is an additional societal burden. Each year, the toll of human suffering extracted by these diseases is overwhelming, with hundreds of millions children and adults world-wide affected.
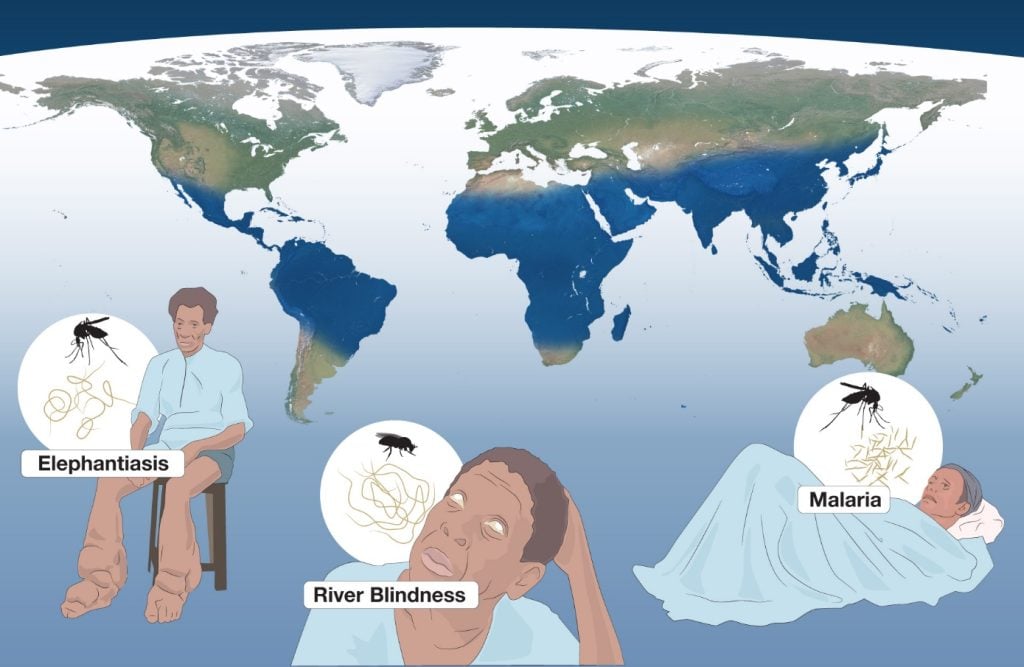
Discovery of Avermectin: A Therapy against Worm Parasites
Intestinal Nematodes
More than one billion people world-wide are infected with one or more species of intestinal nematodes. These diseases hamper economic growth and result in a major health burden which disproportionately affects the poor and are considered diseases of neglected communities and neglected people.
Intestinal nematodes (i.e. ascaris, ancylostoma, trichuris, filaria, onchocerca, strongyloides and loa loa) are roundworms. They vary in length from 1 mm to many centimetres when mature. Their lifecycles are complex and highly varied. Some can be transmitted from person to person, but most require an intermittent host (vector) for transmission. Because most helminth parasites do not self-replicate, repeated exposure to the parasite is required. Hence, clinical symptoms, as opposed to asymptomatic infection, generally develop only with prolonged residence in an endemic area.
River Blindness (Onchocerciasis)
Onchocerciasis or African River Blindness is caused by the filarial worm Onchocerca volvulus. The disease is transmitted solely to humans, when a female blackfly introduces O. volvolus third stage filarial larvae onto the skin of the host, where they penetrate into the bite wound. The larvae form nodules in the subcutaneous tissue of the host, where they mature to adult worms. Female adult O. volvolus worms are about twice the size as males, ranging from 33-50 cm long and 270 to 400 µm wide. Adult worms can live up to 18 years. After mating in the infected host, the female adult worm can release up to 1,000 embryonic microfilariae each day, which subsequently migrate through the body and cause a variety of conditions including chronic dermatitis, skin rashes, lesions, intense itching, and skin depigmentation. Microfilariae reaching the eye cause inflammation, corneal scarring and ultimately blindness. River Blindness is one of the world’s second-leading infectious causes of blindness. The flies are predominantly localized to rivers, hence the name “River Blindness”. The vast majority of Onchocerciasis infections occur in sub-Saharan Africa, south Asia, and South and Central America, afflicting many of the world’s most vulnerable citizens. An estimated 25 million individuals are infected and more than 300,000 suffer from blindness. The total population at risk for onchocerciasis in 31 endemic countries will reach ~250 million by 2016.
Lymphatic Filariasis
Lymphatic Filariasis is an infection of the lymphatic system caused by infection with nematodes of the family Filariodidea (Wuchereria bancrofti; Brugia malayi; Brugia timori). Filarial nematodes carry out their life cycle in two hosts, where humans serve as the definitive host and mosquitoes as their intermediate host. Female nematodes of the Filariodidea family are larger than males. Brugia malayi are smaller than Wuchereria bancrofti, with adult females 43-55 mm in length and 130 to 170 µm wide. Fertilized female nematodes release microfilariae that are 177-230 µm long and 5-7 µm wide and survive for 3-36 months. Macrofilariae typically live five to seven years and reside in the lymphatic system of the human host. Microfilariae migrate in a nocturnal pattern between the deep veins during the day and the peripheral circulation during the night. The life-cycle continues as another mosquito bites the host and becomes infected. Microfilariae then develop into infectious larvae in several stages inside a mosquito’s midgut, which are transferred to a new victim when the next host is bitten. As the number of nematodes in the human body increases over time, the worst effects of this chronic inflammatory disease are felt during adulthood, when the damaged and dilated lymphatic vessels cause blockages, swelling of the limbs, fever and immobilization. Lymphatic Filariasis affects more than 120 million people. It causes chronic swelling and leads to life-long stigmatizing and disabling clinical symptoms including Elephantiasis (Lymphedema) and Scrotal hydrocele.
Discovery of a Microorganism with Unusual Anti-Microbial Properties
Satoshi Ōmura is trained as a microbiologist with expertise in isolating natural products. He worked at the Kitasato Institute in Japan, which had a strong track record in natural product isolation. In 1971, Ōmura went to the United States as a visiting research scientist in the laboratory of Max Tishler at Wesleyan University (Campbell, 2012). Tishler was former director of research at Merck Shape and Dome Research Laboratories (MDRL) and he was a catalyst for setting up a meeting between Ōmura and MDRL, who had a long-standing interest in antibiotic research dating back to the first antibiotics. In 1972, Merck’s chief microbiologist, H. Boyd Woodruff travelled to Kitasato Institute in Japan to establish collaboration for discovering new antimicrobial products from microbial fermentation with Ōmura (Woodruff, 2014). Both Tischler and Woodruff had previously worked with Selman Waksman.
Ōmura focused on a group of bacteria, Streptomyces, which is found in the soil and known to produce a plethora of antibacterial activities (for example Streptomycin discovered by Selman Waksman). While it was appreciated that new strains of Streptomyces were yet to be discovered, this group of bacteria was also notoriously difficult to culture in the laboratory, which hampered progress in this field. Equipped with extraordinary skills in developing unique methods for large-scale culturing and characterization of these bacteria, Ōmura isolated and characterized new strains of Streptomyces from soil samples and successfully cultured these strains in the laboratory (Figure 2) (Ōmura, 2011). From originally several thousands of cultures, he selected around 50 of the most promising for producing novel secondary metabolites, with the intent that they would be further analyzed for antimicrobial activities. One of these new Streptomyces strains, found in soil nearby a golf course in Ito, Japan, would turn out to be the strain (Streptomyces avermitilis) producing Avermectin, (Burg et al., 1979). This strain, was also later called Streptomyces avermectinius.
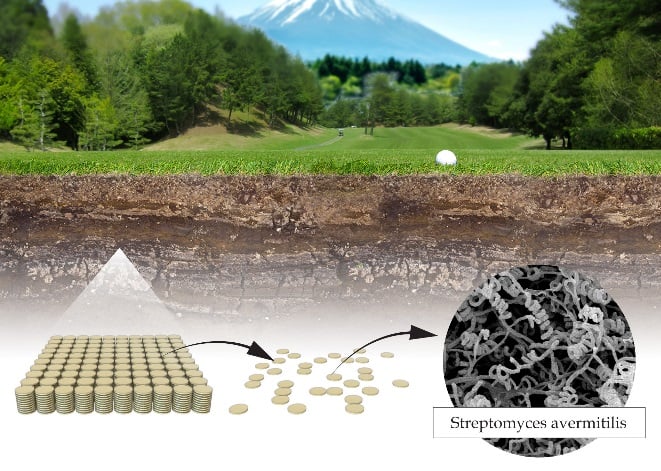
Discovery of the Antiparasitic Activity of Avermectins and Characterization of the Effective Component
William C. Campbell is an expert in parasite biology and was working at MDRL. Under the agreement with the Kitasato Institute he acquired bacterial broths from Ōmura’s cultures and explored their efficacy (Egerton et al., 1979; Campbell, 2012). In a first line of screening, lyophilized broths were mixed in the food and tested in mice with parasite infection to investigate whether the parasites could be reduced. Positive results from one of the cultures were obtained in a mouse infected with Nematospiroides dubius, although the mouse nearly died. This led Ōmura to refine the culturing conditions so that the toxicity from contaminating oligomycin could be weaned out of the preparation (Ōmura, 2011). Thomas W. Miller, working closely with Campbell, chemically characterized the effective components of the preparation, in particular Avermectin B1 (Avermectin) (Miller et al., 1979). Campbell subsequently identified the antiparasitic activity of these agents and demonstrated activity against a variety of parasitic worms in domestic and farm animals (Figure 3) (Blair and Campbell, 1980; Campbell et al., 1979; Klei et al., 1980). Ōmura subsequently applied novel techniques in molecular biology, chemistry, and microbiology to determine the taxonomic status of the organism and later proposed the name Streptomyces avermectinius, based on genetic studies in which the sequence of the genome was established (Ikeda et al., 1987, 1999, 2001 and 2003).
From Avermectin to Ivermectin and Clinical Efficacy
Campbell, together with colleagues at MDRL, subsequently chemically modified Avermectin to a new version called Ivermectin, which turned out to be even more effective against parasitic infection (Chabala et al., 1980). Ivermectin is a semisynthetic version of Avermectin B1 containing two hydrogenation modifications. Thereafter, Campbell initiated a team effort demonstrating that the Ivermectin molecule was: (1) extraordinarily potent; (2) active against many intestinal nematodes in various host species; (3) showing durable long-term activity against some extra intestinal parasites including blood-dwelling filarial microfilariae; (4) active against benzimidazole-resistant nematodes (suggesting a different mode of action); and (5) well-tolerated by the host species, suggesting a good margin of safety (Campbell et al., 1983). Inspired by these positive results from animal studies, especially for reducing dog heartworm, Campbell proposed in 1977, that the Avermectin class be tested for use in humans with parasitic infections causing River Blindness. In 1981-1982, Dr. Mohammed Aziz at MDRL, an expert in River Blindness, conducted the first successful human trial (Aziz et al., 1982). The results were clear – patients given a single dose of Ivermectin showed either complete elimination or near elimination of microfilaria-load, while the adult parasites were untouched. Microfilaria in blood were also effectively eliminated in patients infected with Wuchereria bancrofti, the cause of lymphatic filariasis, after a single dose treatment (Ottesen and Campbell, 1994).
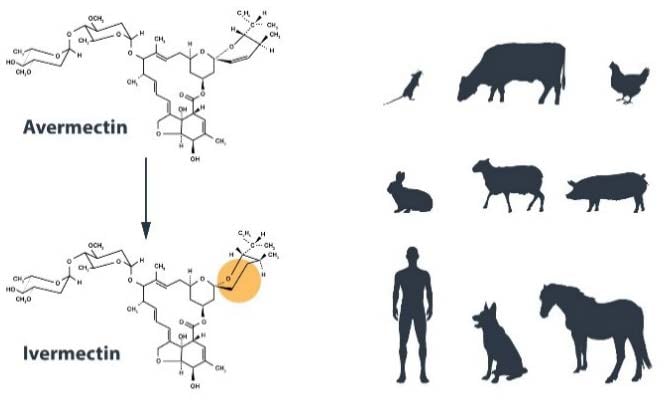
The mode of action of Ivermectin is not known in detail, but involves inhibition of glutamate-gated chloride ion channels (Campbell et al., 1983), and GABA-activated chloride channels (Wang and Pong, 1982) in invertebrate muscle and nerve cells of the microfilaria. This inhibition increases permeability of the cell membrane to chloride ions and results in hyperpolarization of the cell, leading to muscular paralysis and death of the parasite. This effect results in slow death of the parasite, which minimizes inflammation to the host, and alleviates the clinical symptoms of the disease. These specific receptors are present in nematodes, insects and arachnids, but not in cestodes and trematodes (Campbell et al., 1983). These ligand-gated chloride channels are also partly expressed in the mammalian central nervous system, but they have a low affinity and are protected by the blood brain barrier. Thus, Ivermectin is a very selective drug that kills the parasite at low concentrations without harming the host.
Today, Ivermectin is considered a highly effective treatment that only needs to be taken once or twice a year. In this way, citizens of even the most remote parts of the world can be reached. Credit should be given to Dr. Roy Vagelos, CEO at Merck, for influencing the company to make needed quantities of Ivermectin freely available to governments and patients for the treatment of River Blindness and Lymphatic Filariasis (Useem, 1998). The elimination of River Blindness and Elephantiasis are key targets for the WHO Regional Strategy to Eliminate Neglected Tropical Diseases.
As of 2012, over 200 million people received Ivermectin for periods ranging from 1 to 25 years (WHO, 2013). For the elimination of Lymphatic Filariasis 118 million people have received Ivermectin and albendazole for periods ranging from 1 to 11 years (Ichimori, 2014). The global targets for the elimination of Lymphatic Filariasis (2020) and Onchocerciasis, (2025) are within reach (WHO, 2013). Together, the unique contributions by Ōmura and Campbell have led to the identification of a new class of drugs with extraordinary efficiency against parasitic diseases.
Malaria: A global Threat
Malaria is a contagious disease with deep historical roots described in the early Egyptian and Greek literature from ~2000 BC, as well from ancient Chinese writings. Malaria has affected human survival for as long as we know, exerting evolutionary pressure on our genome, such that different populations have evolved with different protective genetic genotypes. For example, people with genetic markers for Sickle cell Disease and Thalassemia, two inherited blood disorders causing severe anemia, are more frequently identified in geographical distributions with a high incidence of malaria. The name Malaria is derived from Medieval Italian, referring to “mal aria” or bad air, stemming from ancient Roman thoughts that malaria came from the pungent fumes of the swamps. Malaria is caused by the single-cell parasite Plasmodium. Five species can infect humans, leading to recurrent attacks of shivering, fever, and sweating. Death and cerebral malaria, a serious condition involving encephalopathy, are usually caused by P falciparum, while the other species usually cause milder forms of malaria. The disease is transmitted by mosquitoes (Anophele). When an infected female mosquito bites a human, it introduces a sporozoite form of the malaria organism into the blood. The sporozoite invades the liver cells, where it reproduces into thousands of asexual merozoites. The liver cells are then ruptured, releasing merozoites into the blood stream and infecting red blood cells. The red blood cell is destroyed by this process, which releases many merozoites that go on to infect new blood cells. In addition, sexual forms of the parasite are taken up by blood-sucking mosquitoes, thereby continuing the life cycle.
According to the WHO World Malaria Report (WHO, 2014), ~3.4 billion people are at risk of being infected with malaria each year. In 2013 alone, 198 million cases of malaria occurred globally, leading to the death of 584,000 people. The disease burden is heaviest in Africa where ~90% of all deaths occur, mainly in children < 5 years of age, who account for 78% of the deaths.
Discovery of the Anti-Malarial Activity of Artemisinin
The devastating impact of Malaria has spurred intense research efforts during the last centuries, leading to a series of Nobel Prizes in Physiology or Medicine. Ronald Ross, a British army surgeon working in India was awarded the Nobel Prize in 1902* after he discovered malaria is transmitted by mosquitoes. Charles Laveran, a French physician working in an Algerian hospital received the Nobel Prize in 1907* after he discovered the existence of parasites inside the red blood cells of malaria-infected patients. He went on to show that quinine treatment eliminated the parasites from the blood. The Swiss chemist Paul Hermann Müller was awarded the Nobel Prize in 1948* for his discovery of DDT as a contact poison against several arthropods including mosquitoes. Soon thereafter, DDT a highly efficient insecticide, was widely used to exterminate mosquitoes, with the hope of reducing the transmission of the parasite between individuals. Together with the newly developed anti-malarial drug, chloroquine, this approach was initially very effective in reducing the incidence of the disease in many countries. However, after only a few decades, mosquitoes developed resistance towards DDT and concomitantly, serious concerns were raised regarding the impact of the insecticide on the environment. Moreover, the durability of chloroquine was also called into question as resistant strains of P falciparium emerged and spread. Both of these factors contributed to the widespread increase in mortality from malaria during the 1960’s.
At this time, with malaria incidence on the upswing, Tu Youyou and her team, as part of a large national project, embarked upon an effort to develop new malaria therapies. For this she turned to traditional Chinese medicine and searched for recipes that had been used for thousands of years to treat fever. Tu’s group discovered that the plant Artemesia annua, sweet wormwood, was distinguished from others, by the fact that it appeared in several hundred recipes.
They tested an extract from this plant initially and found a substantial inhibition of rodent malaria parasites (Figure 4). However, the activity was inconsistent, showing varied results of 12-40% inhibition in subsequent experiments (Tu et al., 1981; 1982). So again Tu turned to ancient literature and studied recipes by Ge Hong 340 AD. There she found a description detailing an approach to obtain “juice” from the leaves of A. annua using cold water instead of the traditional boiling method. This inspired her to use an alternative cold extraction procedure with ether, rather than a previously used ethanol extraction. Using this low-temperature extraction process, Tu achieved consistent results, killing 100% of the malaria parasites in infected mice and monkeys (Tu et al., 1985). This was a genuine break-through in the discovery of Artemisinin and a turning-point in finding a novel drug to combat malaria (Tu, 1985, 1987; Xiao and Tu, 1984; Sun et al., 1991; Tu, 2004; Klayman, 1985).
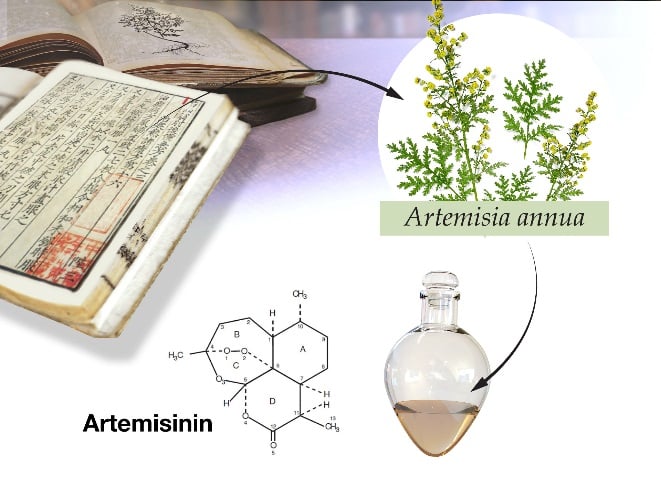
Clinical Efficacy of Artemisinin against Malaria
Encouraged by these experimental findings, Tu undertook clinical tests and found that the plant extract rapidly reduced fever and decreased the number of parasites in the blood of malaria-infected patients. These clinical results were encouraging and inspired Tu’s group to continue their work to isolate the active component in the extract, subsequently called Artemisinin, and later to contribute to efforts in establishing its chemical structure (Tu, 2004; 2011). Artemisinin represents a new class of antimalarial agents that destroy the malaria parasite at an early phase when it infects the red blood cell (Antoine, 2014).
Although the mechanism of action is not entirely known, evidence suggests the Kelch 13 protein is involved. Mutations in the Kelch 13 protein are associated with delayed parasite clearance and are believed to account for an emergence of Artemisinin resistance in some parts of the world (Ariey, 2014). To counteract Artemisinin resistance, in South Asia, but not in Africa, WHO has recommended a combination therapy of Artemisinin and other antimalarial drugs (Artemisinin-based Combination Therapy; ACT).
ACT, in combination with vector control in form of insecticide-treated mosquito nets and indoor residual spraying with insecticides, have dramatically reduced the mortally rate of malaria by 47% between 2000 and 2013 worldwide, and by 54% in Africa (Bhatt et al., 2015). At the same time, the number of malaria infections has dropped 26%, from 173 million to 128 million cases. In children 2-10 years of age, the average malaria infection prevalence has declined from 26% to 14% (WHO, 2014). Today, ACT has profoundly reduced the incidence of malaria, saving millions of lives worldwide.
Tu’s discoveries concerning Artemisinin represent a new class of antimalarial agents that rapidly kill the malaria parasites at an early stage of their development, which explains its unprecedented potency in the treatment of severe malaria.
Avermectin and Artemisinin Therapy – Benefit to Mankind
The discoveries of Avermectin and Artemisinin have fundamentally changed the treatment of parasitic diseases, which primarily affect the most vulnerable people living in marginalized communities. Malaria alone is responsible for the death of 500,000 individuals each year; with the majority (90%) residing in Africa and tragically, 80% are children predominantly under the age of 5.
Artemisinin-based therapy has contributed to the significant reduction in mortality, particularly for children with severe malaria (>30%) (Dondorp et al., 2010). The overall global death toll from Malaria during the last 15 years has declined by 50% (WHO, 2015).
Roundworm infection often occurs during childhood and gives rise to lifelong suffering and disability. Among the multiple diseases caused by roundworm infection, River Blindness and Lymphatic Filariasis stand out, with 25 million and 120 million individuals inflicted for these diseases, respectively. These diseases are included in the Neglected Tropical Diseases, which totally amount to between 46–57 million disability adjusted life-years (DALYs) lost annually. Thus, this group of diseases represents one of the most significant global causes of illness and disability. Africa bears about half of the global health and economic burden of these diseases. Today, treatment is effective, and can be achieved by one or two doses of Ivermectin each year. Ivermectin effectively kills the parasites before they can attack the eyes and the lymphatic system, respectively, and by this, it prevents the disabling symptoms from developing. Moreover, because of its mode of action, Ivermectin is now also considered a preventive medicine.
The global impact of Avermectin and Artemisinin treatment goes far beyond reducing the disease burden of individual people. These parasitic diseases place an enormous disease burden that disproportionately affects the poor and vulnerable. In particular, they place a life-long burden on the inflicted individual by depriving them of the opportunity to gain an education and acquisition of the necessary skill sets to support themselves and their families, further oppressing them and committing them to a life of poverty. Thus, these diseases not only lead to chronic illness, they also are associated with physical and mental disabilities that hamper an individual’s overall health, wellbeing and economic livelihood.
Efforts continue to reduce the transmission of these diseases. Today, River blindness and Lymphatic Filariasis are on the verge of being eradicated. Global initiatives sponsored by WHO and non-governmental organizations (NGOs), as well as Governments, have the goal of eliminating River Blindness by 2025 and Lymphatic Filariasis by 2020. Several countries previously plagued by Malaria are now free from indigenous transmission.
The discoveries of 2015 Nobel Laureates represents a paradigm shift in medicine, which has not only provided a revolutionary therapy for patients suffering from devastating parasitic diseases, but it has also promoted wellbeing and prosperity of both individuals and society. The global impact of their discoveries and the benefit to mankind is immeasurable.
Jan Andersson, Hans Forssberg and Juleen R. Zierath, Karolinska Institutet
References
Antoine, T., Fisher, N., Amewu, R., O’Neill, P.M., Ward, S.A., Biagini, G.A. Rapid kill of malaria parasites by artemisinin and semi-synthetic endoperoxides involves ROS-dependent depolarization of the membrane potential. J Antimicrob Chemother. 69(4):1005-1016, 2014.
Ariey, F., Witkowski, B., Amaratunga, C., Beghain, J., Langlois, A.C., Khim, N., Kim, S., Duru, V., Bouchier, C., Ma, L., Lim, P., Leang, R., Duong, S., Sreng, S., Suon, S., Chuor, C.M., Bout, DM, Ménard, S, Rogers, WO, Genton, B, Fandeur, T, Miotto, O, Ringwald, P, Le Bras, J, Berry, A, Barale, JC, Fairhurst, RM, Benoit-Vical, F, Mercereau-Puijalon, O, Ménard, D. A molecular marker of artemisinin-resistant Plasmodium falciparum malaria. Nature 505(7481):50-55, 2014.
Aziz, M.A., Diallo, S., Diop, I.M., Larivière, M., Porta, M. Efficacy and tolerance of ivermectin in human onchocerciasis. Lancet 2:1456-1457, 1982.
Bhatt, S., Weiss, D.J., Cameron, E., Bisanzio, D., Mappin, B., Dalrymple, U., Battle, K.E., Moyes, C.L.,
Henry, A., Eckhoff, P.A., Wenger, E.A., Briët, O., Penny, M.A., Smith, T.A., Bennett, A., Yukich, J.,
Eisele, T.P., Griffin, J.T., Fergus, C.A., Lynch, M., Lindgren, F., Cohen, J.M., Murray, C.L., Smith, D.L., Hay, S.I., Cibulskis, R.E., Gething, P.W. The effect of malaria control on Plasmodium falciparum in Africa between 2000 and 2015. Nature 16 sept, doi:10.1038, 2015.
Blair, L.S., Campbell, W.C. Suppression of maturation of Dirofilaria immitis in Mustela putorius furo by single dose of ivermectin. J Parasitol. 66(4):691-692, 1980.
Blair, L.S., Campbell, W.C. Efficacy of Ivermectin against Dirofilaria immitis larvae in dogs 31, 60, and 90 days after injection. Am J Vet Res. 41(12):2108, 1980.
Burg, R.W., Miller, B.M., Baker, E.E., Birnbaum, J., Currie, S.A., Hartman, R., Kong, Y., Monaghan,
R.L., Olson, G., Putter, I., Tunac, J.B., Hallick, H., Stapley, E.O., Ruiko O., Omura S. Avermectins, new family of potent anthelmintic agents: producing organism and fermentation. Antimicrob. Agents Chemotherap. 15(3):361-367, 1979.
Campbell, W.C., Blair, L.S., Lotti, V.J. Efficacy of avermectins against Trichinella spiralis in mice. J Helminthol. 53(3):254-256, 1979.
Campbell, W.C., Fisher, M.H., Stapley, E.O., Albers-Schönberg, G., Jacob, T.A. Ivermectin: A potent new antiparasitic agent. Science 221(4613):823-828, 1983.
Campbell, W.C. History of avermectin and ivermectin, with notes on the history of other macrocyclic lactone antiparasitic agents. Curr Pharm Biotechnol. 13(6):853-865, 2012.
Chabala, J.C., Mrozik, H., Tolman, R.L., Eskola, P., Lusi, A., Peterson, L.H., Woods, M.F., Fisher, M.H., Campbell, W.C., Egerton, J.R., Ostlind, D.A. Ivermectin, a new broad-spectrum antiparasitic agent. J Med Chem. 23(10):1134-1136, 1980.
Dondorp, A.M., Fanello, C.I., Hendriksen, I.C., Gomes, E., Seni, A., Chhaganlal, K.D., Bojang, K., Olaosebikan, R., Anunobi, N., Maitland, K., Kivaya, E., Agbenyega, T., Nguah, S.B., Evans, J., Gesase, S., Kahabuka, C., Mtove, G., Nadjm, B., Deen, J., Mwanga-Amumpaire, J., Nansumba, M., Karema, C., Umulisa, N., Uwimana, A., Mokuolu, O.A., Adedoyin, O.T., Johnson, W.B., Tshefu, A.K., Onyamboko, M.A., Sakulthaew, T., Ngum, W.P., Silamut, K., Stepniewska, K., Woodrow, C.J., Bethell, D., Wills, B., Oneko, M., Peto, T.E., von Seidlein, L., Day, N.P., White, N.J. AQUAMAT group. Artesunate versus quinine in the treatment of severe falciparum malaria in African children (AQUAMAT): an open-label, randomised trial. Lancet 376(9753):1647-1657, 2010.
Egerton, J.R., Ostlind, D.A., Blair, L.S., Eary, C.H., Suhayda, D., Cifelli, S., Riek, R.F., Campbell, W.C. Avermectins, new family of potent anthelmintic agents: Efficacy of the B1a component. Antimicrob Agents Chemother. 15(3):372-378, 1979.
Ichimori, K., King, J.D., Engels, D., Yajima, A., Mikhailov, A., Lammie, P., Ottesen, E.A. Global programme to eliminate lymphatic filariasis: the processes underlying programme success. PLoS Negl Trop Dis. 8(12):e3328, 2014.
Ikeda, H., Kotaki, H., Omura, S. Genetic studies of avermectin biosynthesis in Streptomyces avermitilis.
J Bacteriol. 169(12):5615-5621, 1987.
Ikeda, H., Nonomiya, T., Usami, M., Ohta, T., Omura, S. Organization of the biosynthetic gene cluster for the polyketide anthelmintic macrolide avermectin in Streptomyces avermitilis. Proc Natl Acad Sci USA. 96(17):9509-9514, 1999.
Ikeda, H., Nonomiya, T., Omura, S. Organization of biosynthetic gene cluster for avermectin in Streptomyces avermitilis: analysis of enzymatic domains in four polyketide synthases. J Ind Microbiol Biotechnol. 27(3):170-176, 2001.
Ikeda, H., Ishikawa, J., Hanamoto A., Shinose, M., Kikuchi, H., Shiba, T., Sakaki, Y., Hattori M., Omura
S. Complete genome sequence and comparative analysis of the industrial microorganism
Streptomyces avermitilis. Nat. Biotechnol. 21:526-531, 2003.
Klei, T.R., Torbert, B.J., Ochoa, R. Efficacy of ivermectin (22,23-dihydroavermectin B1) against adult Setaria equina and microfilariae of Onchocerca cervicalis in ponies. J. Parasitol. 66(5):859-861, 1980.
Klayman, D.L. Qinghaosu (artemisinin): an antimalarial drug from China. Science 228:1049–1055, 1985.
Miller, T.W., Chaiet, L., Cole, D.J., Cole, L.J., Flor, J.E., Goegelman, R.T., Gullo, V.P., Joshua, H., Kempf, A.J., Krellwitz, W.R., Monaghan, R.L., Ormond, R.E., Wilson, K.E., Albers-Schonberg, G., Putter, I. Avermectins, a new family of potent antihelmintic agents: isolation and chromatographic properties. Antimicrob. Agents Chemotherap. 15(3):338-371, 1979.
Omura S. Microbial metabolites: 45 years of wandering, wondering and discovering. Tetrahedron. 67: 6420-6459, 2011.
Ottesen, E.A., Campbell W.C. Ivermectin in human medicine. J. Antimicrob. Chemotherap. 34(2):195-203, 1994.
Sun, X., Xie, S., Long, Z., Zhang, Z., Tu, Y. Experimental study on the immunosuppressive effects of Qinghaosu and its derivatives (in Chinese). Zhongguo Zhong Xi Yi Jie He Za Zhi 11:37–38, 1991.
Tu, Y., Ni, M., Zhong, Y., Li, L., Cui, S., Zhang, M., Wang, X., Liang, X. Studies on the constituents of
Artemisia annua L (In Chinese). Yao Xue Xue Bao 16:366–368, 1981
Tu, Y., Ni, M., Zhong, Y., Li, L. Studies on the constituents of Artemisia annua L. and derivatives of artemisinin (In Chinese). Zhongguo Zhong Yao Za Zhi 6:31–32, 1981.
Tu, Y., Ni, M., Zhong, Y., Li, L., Cui, S., Zhang, M., Wang, X., Ji, Z., Liang, X. Studies on the constituents of Artemisia annua L. (II). Planta Med. 44:143–145, 1982.
Tu, Y., Yin, J., Ji, L., Huang, M., Liang, X. Studies on the constituents of Artemisia annua L. (In Chinese).
Chinese Traditional and Herbal Drugs. 16:200-201, 1985.
Tu, Y. Study on authentic species of Chinese herbal drug Qinghao. (In Chinese) Bulletin of Chinese material medica. 12:2-5, 1987.
Tu, Y. The development of the antimalarial drugs with new type of chemical structure: Qinghaosu and Dihydro Qinghaosu. Southeast Asian J. Trop. Med. Public Health 35:250–251, 2004.
Tu, Y., Yin, J., Ji, L., Huang, M., Liang, X. Studies on the constituents of Artemisia annua L. (III) (In Chinese). Chin. Tradit. Herbal Drugs 16:200–201, 1985.
Tu, Y., Zhu, Q., Shen, X. Studies on the constituents of Young Artemisia annua L. (In Chinese).
Zhongguo Zhong Yao Za Zhi 10:419–420, 1985.
Tu, Y. The discovery of artemisinin (qinghaosu) and gifts from Chinese medicine. Nature Medicine
17:1217-1220, 2011.
Xiao, Y., Tu, Y. Isolation and identification of the lipophilic constituents from Artemisia anomala S. Moore (In Chinese). Yao Xue Xue Bao 19:909–913, 1984.
Useem, M. Roy Vagelos attacks river blindness. In: The Leadership Moment; Times Books, Random House Inc.: New York N.Y., pp.10-42, 1998.
Wang, C.C., Pong, S.S. Actions of avermectin B1a on GABA nerves. Prog Clin Biol Res. 97:373-395, 1982.
WHO 2013. Program for the elimination of neglected diseases in Africa (PENDA). 2016-2025. www.who.int/apoc/en_apoc_strategic_plan_2013_ok.pdf
WHO Global technical strategy for malaria 2016-2030. 2015; ISBN 978 92 4 156499 1. http://apps.who.int/iris/bitstream/10665/176712/1/9789241564991_eng.pdf?ua=1&ua=1
World Malaria Report 2014. ISBN 978 92 4 156483 http://www.who.int/malaria/publications/world_malaria_report_2014/wmr-2014-no-profiles.pdf?ua=1
Woodruff, H.B. Selman, A. Waksman, Winner of the 1952 Nobel Prize for physiology or medicine. Appl Environ Microbiol. 80(1):2-8, 2014.
Jan Andersson, MD, PhD
Professor of Infectious Diseases, Karolinska Institutet
Adjunct Member of the Nobel Committee
Member of the Nobel Assembly
Hans Forssberg, MD, PhD
Professor of Basic and Clinical Neuroscience, Karolinska Institutet
Adjunct Member of the Nobel Committee
Member of the Nobel Assembly
Juleen R. Zierath, PhD
Professor of Clinical Integrative Physiology, Karolinska Institutet
Chairman of the Nobel Committee
Member of the Nobel Assembly
Illustrations: Mattias Karlén
* Footnotes
The Nobel Prize in Physiology or Medicine 1902 was awarded to Ronald Ross “for his work on malaria, by which he has shown how it enters the organism and thereby has laid the foundation for successful research on this disease and methods of combating it”.
The Nobel Prize in Physiology or Medicine 1907 was awarded to Alphonse Laveran “in recognition of his work on the role played by protozoa in causing diseases”.
The Nobel Prize in Physiology or Medicine 1939 was awarded to Gerhard Domagk “for the discovery of the antibacterial effects of prontosil”.
The Nobel Prize in Physiology or Medicine 1945 was awarded jointly to Sir Alexander Fleming, Ernst Boris Chain and Sir Howard Walter Florey “for the discovery of penicillin and its curative effect in various infectious diseases”.
The Nobel Prize in Physiology or Medicine 1948 was awarded to Paul Müller “for his discovery of the high efficiency of DDT as a contact poison against several arthropods”.
The Nobel Prize in Physiology or Medicine 1952 was awarded to Selman A. Waksman “for his discovery of streptomycin, the first antibiotic effective against tuberculosis”.
The Nobel Prize in Physiology or Medicine 2015 was awarded with one half jointly to William C. Campbell and Satoshi Ōmura “for their discoveries concerning a novel therapy against infections caused by roundworm parasites” and the other half to Tu Youyou “for her discoveries concerning a novel therapy against Malaria”.
William C. Campbell was born in 1930 in Ramelton, Ireland. After receiving a BA from Trinity College, University of Dublin, Ireland in 1952, he received a PhD from University of Wisconsin, Madison, WI, USA in 1957. From 1957-1990 he was with the Merck Institute for Therapeutic Research, from 1984-1990 as Senior Scientist and Director for Assay Research and Development. Campbell is currently a Research Fellow Emeritus at Drew University, Madison, New Jersey, USA.
Satoshi Ōmura was born in 1935 in the Yamanashi Prefecture, Japan and is a Japanese Citizen. He received a PhD in Pharmaceutical Sciences in 1968 from University of Tokyo, Japan and a PhD in Chemistry in 1970 from Tokyo University of Science. He was a researcher at the Kitasato Institute, Japan from 1965-1971 and Professor at Kitasato University, Japan from 1975-2007. From 2007, Satoshi Ōmura has been Professor Emeritus at Kitasato University.
Tu Youyou was born in 1930 in China and is a Chinese citizen. She graduated from the Pharmacy Department at Beijing Medical University in 1955. From 1965-1978 she was Assistant Professor at the China Academy of Traditional Chinese Medicine, from 1979-1984 Associate Professor and from 1985 Professor at the same Institute. From 2000, Tu has been Chief Professor at the China Academy of Traditional Chinese Medicine.
Nobel Prizes and laureates
Six prizes were awarded for achievements that have conferred the greatest benefit to humankind. The 12 laureates' work and discoveries range from proteins' structures and machine learning to fighting for a world free of nuclear weapons.
See them all presented here.