Eric Kandel
Biographical
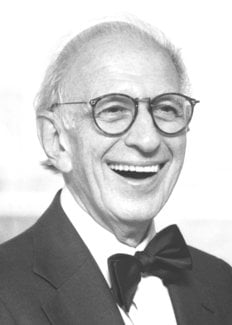
Prologue: Life in Vienna in the 1930s
There was little in my early life to indicate that an interest in biology would become the passion of my academic career. In fact, there was little to suggest I would have an academic career. Rather, my early life was importantly shaped by my experiences in Vienna and I spent many years later coming to grips with the circumstances and place of my birth.
I was born in Vienna on November 7, 1929, eleven years after the multiethnic Austro-Hungarian Empire fell apart following its defeat in World War I. Although Austria had been radically reduced in size (from 54 million to only 7 million inhabitants) and in political significance, its capital, the Vienna of my youth, was still intellectually vibrant, one of the great cultural centers of the world. A city of one and a half million people, it was home to Sigmund Freud, Karl Kraus, Robert Musil, Arthur Schnitzler, and for a while Arnold Schoenberg. The music of Gustav Mahler and of the earlier 19th Century Vienna school resonated throughout the city, as did the bold expressionist images of Gustav Klimt, Oskar Kokoschka, and Egon Schiele. Even as it thrived culturally, however, Vienna in the 1930s was the capital city of an oppressive, authoritarian political system. I was too young to appreciate its cultural richness, but I sensed later, from the perspective of a more carefree adolescence in the United States, the oppressive conditions in Vienna that affected my early youth.
Even prior to the Anschluss in 1938, anti-Semitism was a chronic feature of Viennese life. Jews, who made up nearly 20% of the city’s population, were discriminated against in the Civil Service and in many aspects of social life. Nonetheless, they were fascinated by the city in which they had lived for over a thousand years. My parents genuinely loved Vienna, and in later years I learned from them why the city exerted a powerful hold on them and other Jews. My parents loved the dialect of Vienna, its cultural sophistication, and artistic values. “The greatest grim irony of all was the fierce attachment of so many Jews to a city that through the years demonstrated its deep-rooted hate for them,” wrote George Berkley, the American historian of Vienna and its Jews. This fierce attachment was considered by the historian Harvey Zohn to be the most tragically unrequited love in world history.
In spite of the hostile climate, Austrian Jews continued to make remarkable contributions to theater, music, literature, science, and medicine in the period between the two World Wars. The Salzburg Festival was directed by Max Reinhardt; the Vienna Opera was conducted by Bruno Walter. Stefan Zweig and Franz Werfel were two of the most popular writers in the German language, and Elias Canetti, who later won the Nobel Prize in Literature for books describing his youth in Vienna, began writing these in the 1930s. Two of the three Austrians to be awarded the Nobel Prize in Physiology and Medicine in the 1930s were of Jewish origin: Karl Landsteiner was honored in 1930 for his discovery of blood groups and Otto Loewi in 1936 for discovering acetylcholine, a chemical transmitter that slowed the heart. Of the 52 Olympic medals earned by Austrian athletes from the beginning of modern Olympics to 1936, 18 were won by Jewish Austrians. Fully half of the practicing physicians and medical faculty at the University of Vienna were Jewish. This, in fact, was the last period during which Viennese medicine still attracted students and patients from all over the world. They came to study with, or to be treated by, pioneers in diagnostics and therapeutic medicine, such as the pediatrician Béla Schick, the ear specialist Heinrich von Neumann, and the psychoanalyst Sigmund Freud. As this listing makes clear, the period of my early youth has been characterized, appropriately, as “the final flowering of the Austrian Jewish intellectual activity.”
My parents were not born in Vienna, but they had spent much of their lives there, having each come to the city at the beginning of World War I when they were still very young. My mother, Charlotte Zimels, was born in 1897 in Kolomea, a town of about 43,000 inhabitants in Galicia, a region of the Austro-Hungarian Empire. (Kolomea now is part of the Ukraine and has been renamed Kolomyya.) Almost half the population of Kolomea was Jewish, and the Jewish community had a very active culture. My mother came from a well-educated middle-class family, and although she had spent only one year at the university in Vienna, she spoke and wrote English as well as German and Polish.
My father Herman was born into a poor family in 1898 in Olesko, a small town of about 3,500 people near Lvov (Lemberg), now also part of the Ukraine. During World War I he was drafted into the Austria-Hungarian Army directly from high school. After the war he worked to support himself and never went back to school.
My parents met in Vienna and married in 1923, shortly after my father had established himself as the owner of a small toy store. My brother Lewis was born on November 14, 1924. I was born five years later. We lived in a small apartment at Severingasse 8 in the 9th district, a middle-class neighborhood near the medical school, and not too far from Freud’s apartment, although we had no association with either. Both of my parents worked in the store, and we had a full-time housekeeper to help out at home.
I went to a school near our house. As with most elementary schools in Vienna, it was very traditional and very good, and I followed the well-trodden trail that my exceptionally gifted brother Lewis had blazed five years earlier in the same school with the very same teachers. Throughout my years in Vienna I felt that his was an intellectual virtuosity that I would never match. By the time I began reading and writing, he already was starting to master Greek and to play the piano.
My fondest early memories are of family get-togethers and vacations. On Sunday afternoons my Aunt Minna (my mother’s sister) and Uncle Srul would come for tea. This was an occasion for my father and uncle to play cards, games at which my father excelled and which brought out great animation and humor in him. We celebrated Passover in a festive way at the home of my grandparents Hersch and Dora Zimels, and we invariably went on vacation in August to Monichkirchen, a small farming village in the southeast portion of Lower Austria, not far from Vienna.
It was just as we were about to depart for Monichkirchen in July of 1934 that the Austrian Chancellor, Engelbert Dollfuss, who had outlawed the Nazi Party, was assassinated by a band of Austrian Nazis disguised as policemen – the first political storm to register on my slowly maturing political awareness. Following the Dollfuss assassination and during the early years of the chancellorship of his successor, Kurt von Schuschnigg, the Austrian Nazi Party went further underground, but it continued nonetheless to gain new adherents, especially among teachers and other civil servants. Paradoxically, the Austrian drive toward authoritarianism was abetted by Dollfuss’s own political attitudes and actions. Modeling himself on both Mussolini and Hitler, Dollfuss renamed his Christian Socialist Party the Fatherland Front, and took to wearing a modified swastika. To assure his own control of the government he abolished Austria’s Constitution and outlawed not only the Nazi Party but all opposition parties. Thus, although Dollfus opposed the efforts of the Austrian National Socialist movement to form a Pan-German state with Germany, his abolition of the Constitution and of other political parties helped open the door for Hitler to march in.
And, as I well remember, march in he did. Since his youth, Hitler had dreamed of the union of Austria and Germany. It is therefore not surprising that a key point in the Nazi program, from its beginning in the 1920s, was a merger of all German-speaking people into a Greater Germany. In the fall of 1937 Hitler began to act on this program by raising the level of rhetoric and threatening to move against Austria. Schuschnigg, eager to assert Austria’s independence, met with Hitler on February 12, 1938 in Berchtesgaden. Hitler showed up with two of his generals in tow and threatened to invade Austria unless Schuschnigg, lifted the legal restrictions on the Austrian Nazi Party and appointed three Austrian Nazis to key ministerial positions in the Austrian Cabinet. Schuschnigg refused, but as Hitler continued to intimidate him, Schuschnigg compromised and agreed to a legalization of the Nazi party and to granting it two cabinet positions. The agreement between Schuschnigg and Hitler so emboldened the Austrian Nazis that they began to challenge the Austrian government in a series of incidents that the police had difficulty controlling. Faced with Hitler’s aggression from without and the Austrian Nazi rebellion from within, Schuschnigg took the offensive on March 9th and boldly called for a plebiscite on Austria’s autonomy to be held four days later, on March 13th.
This courageous move caught Hitler by complete surprise, an awkward surprise since it seemed almost certain that the vote would favor an independent Austria. Hitler responded by mobilizing troops and threatening to invade Austria unless Schuschnigg postponed the plebiscite, resigned as chancellor, and formed a new government with an Austrian Nazi, Arthur Seyss-Inquart as chancellor. Schuschnigg turned for help to England and Italy, two countries that had formerly supported Austrian independence. But on this occasion both countries failed him and did not respond. Abandoned by his potential allies and concerned about needless bloodshed, Schuschnigg resigned on the evening of March 11th. “Austria is yielding to force,” he announced in an emotional farewell radio address to the nation. “God protect Austria.” Even though Schuschnigg had resigned and President Miklos of Austria gave in to all the German conditions Hitler nonetheless invaded Austria.
Hitler’s triumphal march into Vienna and his overwhelming reception by the Viennese public made an indelible impression on me. My brother had just finished building his first short-wave radio receiver, and on the evening of March 13th we both were listening with earphones as the broadcaster described the earlier crossing of the Austrian border by German troops on the morning of March 12th. Hitler followed later in the afternoon of that day, crossing the border first at Braunnau am Inn, his native village, and then moving into Linz, the capitol of Upper Austria, where people welcomed him in the marketplace as their native son, screaming “Heil Hitler.” Of the 120,000 people of Linz, almost 100,000 came out to greet Hitler. In the background the Horst Wessel song, one of the hypnotic Nazi marching songs that even I found captivating, blared forth. On the afternoon of March 14th Hitler’s entourage reached Vienna, where a wildly enthusiastic crowd welcomed him as the hero who had unified the German-speaking people.
The extraordinary reception in Linz and Vienna caused Hitler to change his plan. He now realized the Austrians would not demand the status of a relatively independent protectorate of Germany he had planned for them. The enthusiastic welcome convinced him that Austria would readily accept, indeed would welcome, total annexation. For it seemed as if everyone, from the modest shopkeepers to the most elevated members of the academic community, now embraced Hitler. In a shocking move, even Theodor Cardinal Innitzer, the influential Archbischop of Vienna, welcomed Hitler and ordered all the Catholic churches in the city to fly the Nazi flag and to ring the church bells in honor of Hitler’s arrival in Vienna. As the Cardinal personally greeted Hitler, he assured him of his own loyalty and that of all Austrian Catholics – which was most of the population of Austria. The Cardinal promised Hitler that Austria’s Catholics would become “the truest sons of the great Reich into whose arms they had been brought back on this momentous day,” provided that the liberties of the Church were respected and its role in the education of the young guaranteed.
That night, and for days on end, all hell broke loose. Viennese mobs erupted in nationalistic fervor, expressed by beating up Jews and destroying their property. Foreign commentators, long accustomed to Nazi tactics in Germany, were astonished by the wanton brutality of the Austrian Nazis, and even German Nazis were amazed and emboldened by the viciousness of the attacks in Vienna.
In his autobiography the German playwright Carl Zuckmayer, who had moved to Austria in 1936 to escape Hitler, described Vienna during the days following the annexation of Austria as a city transformed “into a nightmare painting of Hieronymus Bosch.” It was as if:
… “Hades had opened its gates and vomited forth the basest, most despicable, most horrible demons. In the course of my life I had seen something of untrammeled human insights of horror or panic. I had taken part in a dozen battles in the First World War, had experienced barrages, gassings, going over the top. I had witnessed the turmoil of the post-war era, the crushing uprisings, street battles, meeting hall brawls. I was present among the bystanders during the Hitler Putsch in 1923 in Munich. I saw the early period of Nazi rule in Berlin. But none of this was comparable to those days in Vienna. What was unleashed upon Vienna had nothing to do with seizure of power in Germany … What was unleashed upon Vienna was a torrent of envy, jealousy, bitterness, blind, malignant craving for revenge. All better instincts were silenced … only the torpid masses had been unchained … It was the witch’s Sabbath of the mob. All that makes for human dignity was buried.”
Having watched the build-up of anti-Jewish laws in Germany following Hitler’s rise to power in 1933, my parents did not need much convincing to realize that the violence at the time of the annexation was not likely to fade away. We knew that we had to leave – and to leave as soon as possible. My mother’s brother, Berman Zimels, had emigrated a decade earlier to New York and established himself as an accountant. He provided us expeditiously with affidavits that assured he would support us upon our arrival in the United States. Even with these affidavits it took about a year for my parents’ Polish quota number to be called. When our number finally was called, we had to emigrate in stages because of United States immigration laws. My mother’s parents left first in February 1939, my brother and I next in April 1939, and finally my parents in September 1939, only days before World War II broke out.
During the one year that we lived under Nazi rule, we experienced directly Vienna’s humiliating form of anti-Semitism. The day after Hitler marched into Vienna, every one of my non-Jewish classmates – the entire class with the exception of one girl – stopped talking and interacting with me. In the park where I played I was taunted and roughed up. This viciousness toward Jews, of which my treatment was a mild example, culminated in the horrors of Kristallnacht, November 8, 1938. On the morning of November 7, 1938, a 17 year-old Jewish youth, who was distraught over his parent’s tragic fate at the hands of the Nazis, shot a third secretary in the German Embassy in Paris, mistaking him for the German Ambassador. In retaliation for this single act, almost every synagogue in Germany and Austria was set on fire. Of all the cities under Nazi control, the destructiveness in Vienna on Kristallnacht was particularly wanton. Jews were taunted and brutally beaten, expelled from their businesses, and temporarily evicted from their homes so that both could be looted by their neighbors. My father was rounded up by the police together with hundreds of other Jewish men. He was released a few days later only because he had fought in the Austria-Hungarian army as a soldier in World War I. I remember Kristallnacht even today, more than 60 years later, almost as if it were yesterday. It fell two days after my ninth birthday, on which I was showered with toys from my father’s shop. When we returned to our apartment a week or so after having been evicted, everything of value was gone, including my toys.
My last year in Vienna was, in a way, a defining year, and it fostered the profound sense of gratitude I came to feel for the life I have led in the United States. It is probably futile, even for someone trained in psychoanalytic thinking as I am, to attempt to trace the complex interests and actions of my later life to a few selected experiences of my youth. Nevertheless I cannot help but think that the experiences of my last year in Vienna helped to determine my later interests in the mind, in how people behave, the unpredictability of motivation, and the persistence of memory. Over the years I have returned to these subjects repeatedly as my professional interests evolved from a youthful interest in European intellectual history at Harvard, where I studied the motivation of German intellectuals during the Nazi era, to an interest in psychoanalysis with its more systematic approach to mental processes, and finally to my interests in the biology of conscious and unconscious memory.
My early experiences in Vienna almost certainly contributed to my curiosity about the contradictions and complexities of human behavior. How are we to understand the sudden release of such great viciousness in so many people? How could a highly educated and cultured society, a society that at one historical moment nourished the music of Haydn, Mozart, and Beethoven, in the next historical moment sink into barbarism?
Clearly the answer to this question is complex, and many scholars of this period have attempted partial answers. One conclusion, troubling to an academic like myself, is that a society’s culture is not a reliable indicator of its respect for human life. This rather simplistic conclusion, of course, raises the question: How can values within a society become so radically dissociated? As far as I can tell, the Viennese achieved this dissociation by shifting their frame of reference. By defining Jews in racial rather than religious terms, they were able to exclude Jews from the “more highly evolved European Aryan race,” the race they believed to be responsible for the rise of Western civilization.
My last year in Vienna was likely also an important factor in my more specific later interest in the mechanisms of memory. I am struck, as others have been, at how deeply these traumatic events of my childhood became burned into memory – and I would emphasize that my experiences were trivial compared to those of so many who were seriously harmed or killed. For me, the frightening experiences of my last year in Vienna are certainly the most powerful of my “flashbulb memories,” the emotionally charged and vivid memory of significant events that came to fascinate me.
Resettlement in the United States
Needless to say, arriving in the United States in April of 1939 was like a breath of fresh air. I never actually said “free at last,” but I felt it then and have ever since. We settled in Brooklyn and lived at first with my mother’s parents. My grandfather Hersch Zimels was a religious and scholarly man who was somewhat unworldly. My brother said that my grandfather was the only man he knew who could speak seven languages but could not make himself understood in any one of them. My grandfather and I liked each other a great deal, and he readily convinced me that he should tutor me in Hebrew during the summer of 1939 so that I might be eligible for a scholarship at the Yeshiva of Flatbush, an excellent Hebrew parochial school that offered both secular and religious studies at a very high level. With his tutelage I entered the Yeshiva in the fall of 1939. By the time I graduated in 1944 I spoke Hebrew almost as well as English, had read through the five books of Moses, the books of Kings, the Prophets and the Judges in Hebrew, and also learned a smattering of the Talmud.
After my parents arrived, my father worked in a toothbrush factory. Even though he was not fond of working in this factory, he threw himself into the work with his usual energy and was soon reprimanded by the union steward for producing toothbrushes too quickly and making other workers appear slow. My father was undeterred. He simply loved America – he often referred to it as the “goldene Medina,” the golden state. Even while still in Vienna he had read avidly the novels of Karl May, an author whose books celebrated the conquest of the American West and the bravery of the American Indians.
With time my father managed to save enough money to rent and outfit a modest clothing store at 411 Church Avenue in Brooklyn. We lived in an apartment above the store. My father and mother worked together and sold simple women’s dresses and aprons, and men’s shirts, ties, underwear, and pajamas. In this way my parents earned enough not only to support us all but also to send me to college and medical school. My father worked in that store until the week before he died at age 78 in 1976. My mother sold the store soon thereafter and died in 1991 at age 94.
Erasmus Hall High School and Harvard College
In 1944, when I graduated from the Yeshiva of Flatbush elementary school, it did not as yet have a high school. I went instead to Erasmus Hall High School, a local public high school in Brooklyn that was then academically very strong. Here I became interested in history, in writing, and in girls. I worked on the school newspaper and became sports editor. I also played soccer and was co-captain of the track team. At the urging of one of my history teachers, John Campagna, a Harvard alumnus, I applied to Harvard College and was one of two students out of my class of about 1,400 to be admitted, both of us on scholarships! Fair Harvard indeed!
Even though I was thrilled by my good fortune, I was apprehensive about leaving Erasmus, convinced that I would never again feel the sheer joy I had experienced there. It was at Erasmus that I first sensed myself emerging from behind the shadow of my brother Lewis. I now had distinctive interests of my own – jazz music, sports, American constitutional history – things that did not interest Lewis. At Harvard I majored in 19th and 20th century European history and literature and wrote my honors dissertation on The Attitude Toward National Socialism of Three German Writers: Carl Zuckmayer, Hans Carossa, and Ernst Junger. Each of these writers was then still alive and represented a different position on the political spectrum of fascism – uncompromising liberal opposition and emigration (Zuckmayer), resigned acceptance and internal (spiritual) emigration (Carossa), and intellectual support (Junger). I came to the rather depressing conclusion that many German artists, intellectuals, and academics succumbed all too eagerly and opportunistically to the nationalistic fervor and racist propaganda of National Socialism. Historical studies have found that Hitler did not have widespread popular support in his first year in office. Had intellectuals mobilized effectively and brought along segments of the general population, Hitler’s government might well have been toppled.
I originally thought of doing graduate work in European intellectual history, along the lines of my undergraduate dissertation. However, in the course of my studies at Harvard I befriended a fellow student, Anna Kris, who had also emigrated from Vienna with her parents, Ernst and Marianne Kris, both prominent psychoanalysts from Freud’s circle. Anna and her parents were very influential in getting me interested in psychoanalysis. It is difficult to recapture now the extraordinary fascination that psychoanalysis held for young people in 1950. During the first half of the 20th century psychoanalysis provided a remarkable set of insights into the mind – insights about unconscious mental processes, psychic determinism, and perhaps most interesting, the irrationality of human motivation. As a result, in 1950, psychoanalysis outlined by far the most coherent, interesting, and nuanced view of the human mind than did any other school of psychology. In addition, Anna’s parents, who represented academic psychoanalysis in its most intellectual and interesting form, were extraordinary people – intelligent, cultured, and filled with enthusiasm. Ernst Kris, a former curator of applied art at the Kunsthistorisches Museum in Vienna, had been a world class art historian before becoming a psychoanalyst. After taking up psychoanalysis, he focused on the psychology of art, an area in which he helped train among others the great historian Ernst Gombrich. Marianne Kris, a wonderful therapist, was the daughter of Oskar Rie, a well-known Viennese pediatrician and Freud’s best friend. Marianne in turn was a close friend of Freud’s distinguished daughter, Anna Freud.
Both Ernst and Marianne Kris were extremely generous and encouraging to me, as they were to Anna’s other friends. As a result of my frequent interactions with them and their colleagues, I was converted to their view that psychoanalysis offered a fascinating new approach, perhaps the only approach, to understanding the mind, including the irrational nature of motivation and unconscious and conscious memory. With time this began to seem much more exciting and interesting to me than European literature and intellectual history.
Medical School at N.Y.U.
To become a practicing psychoanalyst, however, it was best to go to medical school, become a physician, and train as a psychiatrist – a course of study I had not previously considered. So in 1951, almost impulsively, I went to summer school at Harvard and took the required course in introductory chemistry. That summer in Cambridge I shared a house with Robert Goldberger, Henry Nunberg, James Schwartz, and Robert Spitzer, and we all became lifelong friends. A few months later, based on this one chemistry course and my overall college record, I was accepted at N.Y.U. Medical School, with the proviso that I complete the remaining course requirements before I entered medical school in the fall of 1952.
I entered N.Y.U. Medical School dedicated to studying psychiatry and becoming a psychoanalyst. Although I stayed with this career plan through my internship and psychiatric residency, by my senior year in medical school I had become so interested in the biological basis of medical practice (as had everyone else in my class) that I decided I had to learn something about the biology of the mind. In the 1950s most psychoanalysts thought of the mind in nonbiological terms. However, several psychoanalysts – particularly two that I got to know personally and who had a background in neurology, Lawrence Kubie and Mortimer Ostow – had begun to discuss the potential importance of the biology of the brain for the future of psychoanalysis. After considerable discussion with them and with another biologically oriented psychoanalyst, Sydney Margolin, I decided to take an elective period at Columbia University with Harry Grundfest. At that time N.Y.U. had no one on the faculty who was doing basic neural science, and in 1955, Grundfest was the most intellectually interesting neurobiologist in the New York area.
Harry Grundfest’s laboratory at Columbia University
Grundfest had obtained his Ph.D. in zoology and physiology at Columbia in 1930 and went on to a post-doctoral fellowship at Columbia, studying with Selig Hecht, an outstanding psychophysicist interested in phototransduction – the transformation of light into neural signals. (Hecht also was the teacher of George Wald, who won the Nobel Prize in 1967 for discovering the chemical structure of the visual pigments.) Grundfest then joined the Rockefeller Institute in 1935, where he remained for a decade collaborating with Herbert Gasser. In 1944, while Grundfest was in his lab, Gasser shared the Nobel Prize in Physiology or Medicine with Joseph Erlanger for introducing the oscilloscope to neurophysiological studies. This methodology allowed accurate temporal resolution of the waveform and conduction velocities of the propagated action potential. In collaboration with Grundfest, Gasser elaborated on his discovery that the conduction velocity of the action potential is a function of the diameter of the axon. Grundfest also carried out reconstructions of the compound action potential from cross-sectional measurements of axonal diameters in mixed nerves, work that formed much of Gasser’s Nobel Prize Lecture.
In my decision to work with Grundfest, I was strongly encouraged by a new friend, Denise Bystryn, an extremely attractive and interesting French woman I had just met and would later marry. Denise is also Jewish. Her mother helped her father escape from a French concentration camp, and her parents survived the war by hiding from the Nazis in the southwest of France. During a good part of that time Denise was separated from her parents, hidden in a Catholic convent near Cahors. Denise’s experiences, although more difficult, paralleled mine in a number of ways that seemed significant to her but did not seem at all important to me when we first met. However, over the years, our shared experiences in Europe proved to be defining in both our lives.
In 1949, Denise, her brother Jean-Claude, and her parents emigrated to the United States. Denise attended the Lycée Français de New York for one year and was admitted at age 17 to Bryn Mawr College as a junior. On graduating from Bryn Mawr at age 19, she enrolled at Columbia University as a graduate student in sociology. When we met she had just started research for her Ph.D. thesis in medical sociology with Robert Merton. Denise’s father, a gifted mechanical engineer who unfortunately died one year before I met Denise, had advised her to marry a poor intellectual because he would likely be sufficiently ambitious to do interesting scholarship. Denise believed she was following that advice (she certainly married someone who was poor) and always encouraged me to make decisions that favored my doing science.
In Grundfest’s lab I spent the first several months working on a number of projects with Dominick Purpura, an independent young scientist just starting out on his own career of cortical physiology. To my surprise I found my first experience in a lab really interesting, and very different from the classroom. Of course the research questions we were asking fascinated me and the discussions were penetrating and enjoyable. Dominick was very bright and very entertaining. (I have referred to him as the Woody Allen of neurobiology.) But the actual performance of the experiments was also pleasurable and, when successful, very satisfying. Nevertheless, I began to worry about the methods we were using to address rather sophisticated questions about the electrical properties of dendrites. We were using evoked responses that were initiated by stimulating small areas of cortex, thereby activating thousands of neurons, and I thought these methods were too indirect to give easily interpretable results. Grundfest and Purpura, of course, were also concerned and talked repeatedly about doing direct intracellular recordings from cortical neurons, but neither thought this was likely to succeed.
An introduction to Stephen Kuffler
It was in this frame of mind that I was introduced to Stephen Kuffler, a Viennese trained physician turned physiologist, who (together with Bernard Katz and John Eccles) was to become one of my great neurobiological heroes. One evening Grundfest threw into my lap the September 20, 1955 issue of the Journal of General Physiology, with three of Kuffler’s papers on excitation and inhibition in the dendrites and soma of isolated sensory nerve cells of the lobster and crayfish. Grundfest said something about Kuffler’s being very good, and that these papers provided direct evidence for the graded properties of dendrites evidence that was consistent with what he and Purpura were seeing in cortica; neurons. I took the issue home and read the papers as best I could. Although I understood relatively little, one thing stood out immediately. Kuffler was studying the dendrites in a preparation in which he actually saw the dendrites and could record from them directly. For studying dendrites Kuffler used an invertebrate sensory neuron that sent its dendrites into skeletal muscle much like the muscle spindles of vertebrates. In the introduction to the three papers Kuffler wrote:
“The greatest advantage of the present preparation lies in its accessibility, since all cellular components can be isolated and visually observed. Further, the state of excitability of the structures could be controlled and graded by utilizing the physiological mechanisms given by the stretch receptor nature of the preparation…
It seems of special interest that the sensory cell of crustacea possessed numerous anatomical features, which bear a striking resemblance to many central nervous system cells of vertebrates.”
I learned from Kuffler’s papers a new criterion for how good science is done – the importance of having a preparation suitable to testing the questions to be answered. Kuffler taught me to respect the power of invertebrate neurobiology.
On graduating from medical school in June 1956, I married Denise and, after a brief honeymoon in Tanglewood, I started an internship at Montefiore Hospital as she continued her thesis research at Columbia. I returned to Grundfest’s lab, spending six weeks with Stanley Crain, who had pioneered the electrophysiological study of nerve cells in tissue culture. Stanley taught me how to make microelectrodes and how to obtain and interpret intracellular recordings from the crayfish giant axon. These experiments confirmed the insights I had gained from reading Kuffler’s paper. From Stanley I also received my first insights into the universality of cellular processes.
Based on my two brief periods in his laboratory, Grundfest offered to nominate me for a position at the NIH, an alternative to serving in the physician’s draft, which provided medical personnel for the military during the years following the Korean War. On the basis of Grundfest’s recommendation, I was accepted by Wade Marshall, Chief of the Laboratory of Neurophysiology at NIMH/NINCDS.
The laboratory of neurophysiology at the National Institutes of Health
By the time I arrived in Bethesda, Wade Marshall had passed the peak of what had been a remarkable career. In the 1930s he was arguably the most promising and accomplished young scientist working on the brain in the United States. As a graduate student at the University of Chicago in Ralph Gerard’s lab in 1936, he discovered that one could record electrical deflections in the somatosensory processing area of the cerebral cortex by moving the hairs of a cat’s limb. He appreciated that one might use this electrical signal (the evoked response) to map the representation of the body surface on the brain.
To study this further, he joined Phillip Bard, Chairman of the Department of Physiology at the Johns Hopkins Medical School, as a post-doctoral fellow. In 1937, Bard had already established himself as a major presence in American neurophysiology. Together with his student Clinton Woolsey, Bard had surgically removed the somatic sensory cortex of the monkey and studied its effect on the “placing reaction,” a form of tactile behavior. Marshall joined up with Woolsey and Bard and together they carried out a classic series of studies in which they mapped sensory inputs from the body surface in the somatic sensory cortex and showed that a topographical representation of the entire body is wired into the brain. This provided the first systematic view of the neural representation in the brain of a sensory system. Today, this map is still shown in every textbook of neural science. Marshall next collaborated with John Talbott and mapped the retinal inputs in the striate cortex. Finally, with Harlow W. Ades, he mapped the cochlear inputs in the auditory cortex.
With these classic studies Marshall revolutionized the study of the sensory representations in the brain and showed that the brain had systematic topographical maps of the sensory surface for each of the three major sensations – touch, vision, and hearing. These marvelous scientific achievements came at a price, however, leaving Marshall so psychologically fatigued that he collapsed and, for a number of years, left science altogether. When he returned, in about 1945, he moved on to a completely new problem: the study of spreading cortical depression, a propagating, reversible silencing of cortical electrical activity. Marshall enjoyed doing occasional experiments, but he had lost his scientific drive and now focused much of his energy and interests on administrative matters, which he did well. Although eccentric, moody, and somewhat unpredictable, he was a wonderful lab chief. In particular, he was supportive and generous to young scientists and gave us a great deal of freedom.
Just before I arrived at NIH in 1957, the neurosurgeon William Scoville and the cognitive psychologist Brenda Milner had described the now-famous patient H.M. In order to treat intractable bilateral temporal lobe epilepsy, Scoville had removed on both sides of H.M.’s brain the medial temporal lobes, including a structure deep to them called the hippocampus. As a result of this procedure, H.M.’s seizures were largely eliminated. But, while retaining all cognitive functions, H.M. lost the ability to put new information into long-term memory. These findings pinpointed the medial temporal lobe and the hippocampus as sites specialized for memory storage.
Until the Scoville and Milner paper, the person most identified with attempts to localize memory was Karl Lashley, Professor of Psychology at Harvard and perhaps the dominant figure in American neuropsychology in the first half of the 20th century. Lashley explored the surface of the cerebral cortex in the rat, and systematically removed different cortical areas. In so doing, he failed to identify any particular brain region that was special to or necessary for the storage of memory. Based on these experiments, Lashley formulated the law of mass action, according to which memory is not localized to any specific region of the cortex but was a distributed property of the cortex as a whole. The extent of any memory defect, Lashley argued, was correlated with the size of the cortical area removed, not with its specific location.
Since I had already begun to think about problems in psychiatry and psychoanalysis in biological terms, the cell and molecular mechanisms of learning and memory struck me as a wonderful problem to study. I had first become interested in the study of learning at Harvard, where B.F. Skinner, the great behaviorist, was a dominant force in the 1950s. It was clear to me even then that learning and memory were central to behavior, and thus to psychopathology and to psychotherapy. Nothing was known about the cellular mechanisms of learning and memory, and now the cellular techniques for studying them were just becoming available – some beginnings of which I had learned from Stanley Crain in Grundfest’s lab.
My initial ideas about how to tackle the biology of memory upon arrival at the NIH were confused and vague. Because intracellular recordings seemed to me such a powerful analytic tool for studying nerve cells, and because the hippocampus seemed particularly important for memory, I wanted to explore the hippocampus in cellular terms. This was made even more attractive for me because, as the great Spanish anatomists Ramón y Cajal and Lorente de Nó had pointed out, the cellular architecture of the hippocampus is remarkably conserved among mammals, and the main cell type, the pyramidal cell, is found in a discrete layer that is easy to target with microelectrodes. In addition, the pyramidal cells send their axons into a large fiber tract (the fornix), which allows the pyramidal cells to be identified electrophysiologically by stimulating the axons in the fornix and backfiring the pyramidal cells. I thought it would be interesting to compare the pyramidal neurons to the only other mammalian neurons that had been well studied at that time – the motor neurons of the spinal cord. I had the idea that the properties of the pyramidal cells themselves might reveal something about memory storage. I was emboldened to try this technically demanding study because Karl Frank was in the laboratory next to ours, pioneering the examination of spinal motor neurons with intracellular recordings in parallel with John Eccles. Although Frank himself thought that studying the hippocampus was chancy, he was not discouraging.
Almost as soon as I began, my research took an extremely fortunate turn in the person of Alden Spencer, who arrived in Marshall’s lab having just graduated from the University of Oregon Medical School. Like me, Alden was becoming interested in the biology of learning and memory. It therefore took little effort for me to convince him that we should join forces on the hippocampus. Although Alden had no experience with intracellular recordings, he had done electrophysiological research on the brain at the University of Oregon Medical School, where he worked with John Brookhardt. Among Alden’s many remarkable talents, he had good surgical skills and a fine knowledge of the anatomical organization of the mammalian brain.
Being both naïve and brash, we were not reluctant to tackle what appeared to Frank and others to be technically difficult problems, namely obtaining intracellular recordings from cortical neurons in a pulsating brain. Alden and I developed a simple way of reducing pulsations in the hippocampus that allowed us to obtain, on occasion, high-quality recordings for a long enough period – up to one hour – to carry out an initial analysis of the electrical properties of the hippocampal pyramidal cells. By applying to the hippocampus the powerful methodologies we learned from Frank, we easily picked some low-lying intellectual fruit. First, we found that action potentials in hippocampal neurons were initiated not only at the axon hillock, as in motor neurons, but also at a second site, which we inferred to be the apical dendrites. These putative dendritic action potentials, which we called fast prepotentials, appeared to trigger the firing at the axon hillock. Second, we found that the hippocampal neurons, unlike motor neurons, were not silent in the absence of synaptic activity, but tended to fire spontaneously, and that this firing often took the form of bursts of spikes that were maintained by summation of depolarizing afterpotentials. Third, we found that the hippocampal neurons engaged a powerful recurrent inhibitory system that gave rise to a prolonged inhibition – several orders of magnitude longer than the inhibition seen in the spinal cord.
The mere technical success of obtaining intracellular recordings from hippocampal neurons, and the few interesting questions we were able to address, caught the enthusiastic attention of, and drew encouragement and help from, our senior colleagues at the NIH – Marshall, Frank, Michael Fuortes, Frank’s gifted colleague and the great Japanese-American biophysicist Ichiji Tasaki. When John Eccles visited the NIH, he also was generous in his comments. But even in our brashest moments, we both realized that ours was a typical NIH story. In the Intramural Program at the NIH, young inexperienced people were given the opportunity to try things on their own, knowing that wherever they turned there were experienced people to help out.
Moreover, as Alden and I reviewed our work we realized that the cellular properties of hippocampal neurons were not sufficiently different from those of spinal neurons to explain the ability of the hippocampus to store memory. Thus, it dawned on us what in retrospect is quite obvious: that the neuronal mechanisms of learning and memory probably did not reside in the properties of the neurons themselves. Rather, because the signaling properties of neurons are quite alike, we began to think that what must matter is how neurons are functionally connected. The basis of learning must reside in the modification of interconnections by appropriate sensory signals. This conclusion, so clear in retrospect, emerged only gradually as we learned, mostly through reading and discussions with one another, to think more effectively about the biology of learning and memory.
This realization led us to reappraise our strategy. Since the hippocampus has a large number of neurons and an immense number of interconnections, it was not the place to begin. Even though we were now quite familiar with the hippocampus, it would be extremely difficult to work out how sensory information specific to learning reached the hippocampus or how learned information processed by the hippocampus might influence motor output.
Alden and I therefore became convinced that to make headway with the study of learning at the cellular level required a very different approach. Alden, a committed mammalian neurophysiologist, turned to the study of the spinal cord, particularly the modifiability of spinal reflexes, and went on to make important contributions in collaboration with Richard Thompson.
However, even the spinal cord proved too difficult for a detailed cellular analysis, and both Alden and Thompson ended up leaving it.
The search for a tractable system for studying learning
Influenced by Kuffler, Grundfest, and Crain, I yearned for a more radically reductionist approach to the biology of learning and memory. I wanted a system that would serve the cellular study of learning as well as the squid giant axon had served for studies of the action potential, or the nerve-muscle synapse of the frog had served for the study of synaptic transmission. I wanted to examine learning in an experimental animal in which a simple behavior was modifiable by learning. Ideally that behavior should be controlled by only a small number of large and accessible nerve cells, so that the animal’s overt behavior could be related to events occurring in the cells that control that behavior.
Such a reductionist approach has been traditional in biology. In neurobiology it is exemplified by the work on the squid giant axon by Hodgkin and Huxley, the nerve-muscle synapse of the frog by Bernard Katz, and the eye of Limulus by Keefer Hartline. When it came to the study of behavior, however, most investigators were reluctant to apply a strict reductionist strategy. In the 1950s and 1960s it was often said that behavior was the area in biology in which simple animal models, particularly invertebrate ones, were least likely to produce fruitful results because the brain that really learns, the mammalian brain, especially the human brain, is so complex that inferences from studies of invertebrates would not stand up. It was thought that humans, because of higher-order capabilities not found in simpler animals, must have types of neuronal organization that are qualitatively different from those found in invertebrates. Although these arguments held some truth, they overlooked certain critical issues. Work by students of comparative behavior, such as Konrad Lorenz, Niko Tinbergen, and Karl von Frisch, had already shown that certain behavior patterns, including elementary forms of learning, were common to humans and simple animals. From the outset I therefore believed that the mechanisms of memory storage were likely to be conserved in phylogeny, and that a cellular analysis of learning in a simple animal would reveal universal mechanisms that are also employed in more complex organisms.
Not surprisingly, I was strongly discouraged in the early days from pursuing this strategy by some senior researchers in neurobiology, particularly John Eccles. His concern reflected, in part, the existing hierarchy of acceptable research questions in neurobiology. Few self-respecting neurophysiologists, I was told, would leave the study of learning in mammals to work on an invertebrate. Was I compromising my career? Of an even greater concern to me were the doubts expressed by some very knowledgeable psychologists I knew, who were sincerely skeptical that anything interesting about learning and memory could be found in a simple invertebrate animal. I had made up my mind, however. Since we knew nothing about the cell biology of learning and memory, I believed that any insight into the modification of behavior by experience, no matter how simple the animal or the task, would prove to be highly informative.
After an extensive search that included crayfish, lobster, flies, and the nematode worm Ascaris, I settled on Aplysia, the giant marine snail. Aplysia offered three major technical advantages: (1) its nervous system has a small number of cells, (2) the cells are unusually large, and, as I realized with time, (3) many of the cells are invariant and identifiable as unique individuals. Before leaving the NIH in 1960, I arranged with Ladislav Tauc, one of the two people in the world then working on Aplysia, to join him in September 1962, as a postdoctoral fellow, as soon as I had completed my residency training. Here again, Denise’s advice was decisive. The only two people working on Aplysia were French – Tauc’s lab was in Paris, and Angelique Arvanitaki-Chalazonitis worked in Marseilles. So far so good! But, Denise, ever the Parisian chauvinist, thought that living in Marseilles would be like living in Albany (a small town in upstate New York). So Tauc and Paris it was, and that proved an excellent choice.
Residency training in psychiatry at the Harvard Medical School
However, before I would leave for Paris I had already committed to a two-year residency training in psychiatry. I therefore left the NIH in the spring of 1960 to start my psychiatric residency at the Massachusetts Mental Health Center of the Harvard Medical School. When I arrived at Harvard, I found an unanticipated bonus. Steven Kuffler, whose thinking had so influenced my own, had been recruited one year earlier from Johns Hopkins to build up neurophysiology at Harvard. Kuffler brought with him several young post-doctoral fellows – David Hubel, Torsten Wiesel, Ed Furshpan, and David Potter – each of whom was extraordinarily gifted. In this way Kuffler succeeded, in one fell swoop, in setting up at Harvard the premier group of neural scientists in the country. I now had my first opportunity to interact with Kuffler and with the remarkable people he had assembled around him. Even though I was in fulltime residency training, Kuffler and his group were extremely accessible, and their generosity allowed me to remain intellectually engaged in neurobiology. Moreover, Jack Ewalt, the Professor of Psychiatry at the Massachusetts Mental Health Center, provided me with funds and space so that I even managed to do some research in my spare time. I obtained the first intracellular recording from hypothalamic neuro-endocrine cells and found that these hormone-releasing cells had all the electrical properties of normal nerve cells.
During my psychiatric residency I began to think about simple forms of learning in preparation for work on Aplysia. I read Kimble’s wonderful revision of Hilgard and Marquis’s classical text Conditioning and Learning, and I reread Skinner’s great book The Behavior of Organisms. This reading made me realize that the paradigms of simple learning articulated by Pavlov and Thorndyke, describing changes in behavior in response to controlled stimulation, included precise protocols for stimulating experimental animals. It occurred to me that the paradigms they described – habituation, sensitization, classical conditioning, and operant conditioning – could readily be adapted to experiments with an isolated Aplysia ganglion using artificial electrical rather than natural sensory stimuli. While recording the behavior of a single cell in a ganglion, one nerve axon pathway to the ganglion could be stimulated weakly electrically as a conditioned stimulus, while another pathway was stimulated as an unconditioned stimulus, following the exact protocol used for classical conditioning with natural stimuli in intact animals. One could then see whether synapses changed systematically in response to these patterns of stimulation, and, if so, whether the synaptic changes in any way paralleled changes in the overt behavior of intact animals, which classical psychologists had described. It thus dawned on me that in this way one could begin to take an initial step toward the study of learning in the intact animal by analyzing what I soon began to call analogs of learning – higher-order stimulus sequences based on patterns of stimulation used in learning experiments in intact behaving organisms, but applied directly to a neuronal system.
Paris, Aplysia, and neural analogs of learning: chemical synapses prove to be remarkably plastic
Based on this idea, I wrote a successful application for an NINCDS postdoctoral fellowship for work to be done in Tauc’s laboratory. And in September 1962, about a year after our son Paul was born, the three of us took off for Paris. Tauc proved an excellent person to work with; both our interests and our areas of competence complemented each other. He was, of course, completely at home with Aplysia, but he also had a strong background in physics and biophysics, which I lacked. Born in Czechoslovakia, Tauc had originally studied the electrical properties of plant cells. As a result, he had no experience with behavior and had up to this point thought little about the problems of neuronal integration that dominated thinking about the mammalian brain – problems that Alden and I had discussed incessantly. Tauc was quite enthusiastic about my approach, which proved even more effective than anticipated. In my cellular studies of analogs of habituation, sensitization, and classical conditioning in Aplysia, I found synaptic changes that paralleled the behavioral changes seen in experiments on intact animals. This encouraged us to write in our 1965 paper in the British Journal of Physiology:
“The fact that the EPSPs (excitatory postsynaptic potentials) can be facilitated for over half-an-hour with an input pattern scheme designed to simulate a behavioral conditioning paradigm, also suggests that the concomitant changes in the efficacy of synaptic transmission may underlie certain simple forms of information storage in the intact animal.”
A brief return to the Harvard Medical School
Upon completing a very productive 16-month stay in Tauc’s laboratory, I returned to Harvard in November 1963. More than a year and a half later, in July of 1965, our daughter Minouche was born, completing our family – one boy, one girl – exactly what we had hoped for.
During this period I struggled with three choices that were to have a profound effect on my subsequent career. First, I realized that to do effective science I could not combine basic research and a clinical practice in psychoanalysis, as I had earlier hoped. I therefore decided not to apply to the Boston Psychoanalytic Institute, a decision which meant that I would not attempt to become a psychoanalyst but devote myself full-time to science. It was my strong sense that one of the problems within academic psychiatry, a problem that has become only worse with time, is that young people take on much more than they can handle effectively. I concluded that I could not and would not do that.
The second choice arose a few months later when Dr. Ewalt and Dr. Howard Hiatt, then chairmen of the Department of Medicine at the Beth Israel Hospital at Harvard, suggested that I take on the newly vacated chairmanship of the Department of Psychiatry at the Beth Israel Hospital. For a moment I was forced to rethink my decision to focus full time on science. The person who had just left that position, Grete Bibring, was a leading psychoanalyst who had been a colleague of Marianne and Ernst Kris in Vienna. Earlier in my life achieving this position would have represented my highest aspiration. But by 1965, my thinking had moved in a very different direction, and I decided against it with Denise’s strong encouragement. (Denise summarized it simply: ‘What?” she said, “throw your scientific career away?”) Instead, I made my third decision. I decided to leave Harvard and accept an invitation to start a small neurophysiology group focused specifically on the neurobiology of behavior in the Departments of Physiology and Psychiatry at the New York University Medical School.
Harvard was quite wonderful, and it was not easy to leave that intellectually heady neurobiology environment. My interaction with Kuffler had increased after my return from Paris and, until his death in 1980, Kuffler proved a marvelous friend and counselor. Moreover, my interactions during this period with members of Kuffler’s group – Hubel, Wiesel, Furshpan, Potter, and Ed Kravitz, a biochemist who joined them later – were extensive and I learned much from them. Many years later, at a small meeting at the Marine Biological Laboratory in Woods Hole in honor of Steve Kuffler, I was surrounded by Steve’s Harvard entourage, some of whom were struggling with the decision of whether to leave Harvard for attractive positions elsewhere. I could not resist beginning my lecture with the remark, “I am here as living proof that there is life after Harvard.”
New York University and a focus on the behavior of Aplysia
The position at N.Y.U. had several great attractions that, in the long run, proved critical. First, it brought us back to New York and closer to my parents and to Denise’s mother, all of whom were having medical problems that benefited from our being nearby. Second, N.Y.U. gave me the opportunity to recruit an additional senior neurophysiologist, and Alden Spencer agreed to move to N.Y.U. from the University of Oregon Medical School where he had returned after his stay at the NIH, and to occupy the laboratory next to mine. Although Alden and I never collaborated experimentally again, we talked daily not only about our science – the neurobiology of behavior – but also about almost everything else, until his untimely early death at age 46 from amyotrophic lateral sclerosis in 1977, when we had already moved to Columbia University. During the period he was alive, no one influenced my thinking on matters of science as much as Alden. I still think about him frequently.
Alden and I arrived at N.Y.U. together in the winter of 1965. Within a year we were joined by a biochemist, James H. Schwartz, whom I had first met in the summer of 1951 at Harvard summer school and who was now a member of the Department of Microbiology at N.Y.U. and was becoming interested in behavior. The three of us formed the nucleus of the Division of Neurobiology and Behavior at N.Y.U.
With several important decisions behind me, I made a strong effort to focus on whole-animal behavior. In France I had found that chemical synapses are remarkably plastic; they could readily undergo long-lasting changes in strength. But I had no evidence that these analogs of learning were in fact behaviorally meaningful. I had no reason to believe that these are the sorts of changes that actually occur when an animal learns something. Although during my last few weeks in France I had begun to replicate my results by substituting natural stimuli for electrical stimulation of nerves, I still had not shown that synaptic plasticity actually occurred during behavioral learning. As a first step I thought it essential to show that Aplysia was capable of learning. With this in mind, I set about recruiting a postdoctoral fellow with a specific interest in behavioral learning. I was fortunate to recruit, first to Harvard and then to N.Y.U., Irving Kupfermann, an extremely critical and thoughtful student of behavior. We were later joined by another learning psychologist, Harold Pinsker, and together we set about delineating a very simple behavior that we could study: the gill-withdrawal reflex. We quickly found that this simple reflex could readily be modified by two forms of learning: habituation and sensitization.
As we explored the two forms of learning, we focused on short-term memory. In 1971, we were joined by another experienced behavioral psychologist, Tom Carew, who brought a new level of energy and insight to our behavioral studies. He arrived as Pinsker was leaving, and soon after we shifted from working with restrained to unrestrained animals, thus opening up the study of long-term memory. Tom found that spaced repetition converted the memory for short-term habituation and sensitization to longer-lasting memories. In 1981, after several unsuccessful attempts, Carew, Terry Walters, Tom Abrams, and Robert Hawkins finally were able to define the conditions for reliably producing classical conditioning in Aplysia. This was a particularly exciting period; Carew, Walters, Hawkins, and I met regularly to discuss how to explore whether a simple reflex, in a simple invertebrate, could show the higher-order cognitive features of classical conditioning recently demonstrated in mammals by Leo Kamin and somewhat later by Robert Rescorla and Alan Wagner. Soon, Hawkins indeed was able to demonstrate that the gill-withdrawal reflex can undergo second-order conditioning, blocking, overshadowing and other cognitive aspects of associative learning, features that were surprising to uncover in such a simple behavior.
We thus were able to describe a rather rich repertory of learning in Aplysia. But long before this inventory of the animal’s behavior was complete, we returned to our initial concerns. What happens in the brain of an animal when it actually learns a task? How does it remember? We proceeded, first with Kupfermann and Vincent Castellucci and then with Jack Byrne and Hawkins, to work out most of the neural circuit of the gill-withdrawal reflex. We identified specific sensory neurons and motor cells that produced movements of the gill. Next, we found that the sensory neurons made direct connections to the motor neurons as well as indirect connections through interneurons, both excitatory and inhibitory. The aversive tail stimuli that produced sensitization of the gill-withdrawal reflex activated modulatory interneurons that acted on terminals of the sensory neurons. We now could turn to think about how learning might occur in this reflex.
Cellular mechanisms of learning
At the end of the 19th century Ramón y Cajal introduced the principle of connection specificity, according to which, during development, a neuron will form connections only with certain neurons and not with others. Kupfermann, Castellucci, and I saw in the circuitry of the gill-withdrawal reflex of Aplysia this remarkable regularity of connections that Cajal referred to and we saw, in exquisite detail, that specific identified cells made invariant connections to one another. But this invariant organization of neurons posed deep questions. How could we reconcile hardwired circuits in the nervous system and the specificity of connections with the animal’s capability for learning? Once acquired, where or how is learned information retained in the nervous system?
One solution was proposed by Ramón y Cajal in his Croonian Lecture to the Royal Society of London in 1894 when he suggested that “… mental exercise facilitates a greater development of the protoplasmic apparatus and of the nervous collaterals in the part of the brain in use. In this way, pre-existing connections between groups of cells could be reinforced by multiplication of the terminal branches of protoplasmic appendices and nervous collaterals.”
This remarkably prescient idea was by no means generally accepted. On the contrary, different theories of learning at various times held the attention of neural scientists. Two decades after Ramón y Cajal’s proposal, the physiologist Alexander Forbes suggested that memory is sustained not by changes in synaptic strength of the sort suggested by Ramón y Cajal, but by dynamic changes resulting from reverberating activity within a closed loop of self-exciting neurons. This idea was elaborated by Ramón y Cajal’s student, Rafael Lorente de Nó, who found in his own and in Ramón y Cajal’s analyses of neural circuitry neurons that were interconnected in closed pathways and could thereby sustain reverberatory activity, thus providing a dynamic mechanism for information storage. In his influential book The Organization of Behavior (1949), D.O. Hebb proposed that a “coincident activity” initiated the growth of new synaptic connections as part of long-term memory storage. But for short-term memory, Hebb invoked a reverberatory circuit:
“To account for permanence, some structural change seems necessary, but structural growth presumably would require an appreciable time. If some way can be found of supposing that a reverberatory trace might cooperate with the structural change, and carry the memory until the growth change is made, we should be able to recognize the theoretical value of the trace, which is an activity only without having to ascribe all memory to it. The conception of a transient, unstable reverberatory trace is therefore useful. It is possible to suppose also some more permanent structural change reinforces it.”
Similarly, in The Mammalian Cerebral Cortex, an influential book of 1958, B. Deslisle Burns challenged the relevance of synaptic plasticity to memory.
“The mechanisms of synaptic facilitation which have been offered as candidates for an explanation of memory … have proven disappointing. Before any of them can be accepted as the cellular changes accompanying conditioned reflex formation, one would have to extend considerably the scale of time on which they have been observed to operate. The persistent failure of synaptic facilitation to explain memory makes one wonder whether neurophysiologists have not been looking for the wrong kind of mechanisms.”
Indeed, some scholars even minimized the importance of specific neuronal connections in the brain, advocating instead mechanisms of learning that were partially or even totally independent of “pre-established” conduction pathways. This view was held by Wolfgang Kohler and the famous Gestalt psychologists, and subsequently by the neurophysiologists Ross Adey and Frank Morrell. Thus, in 1965, Adey wrote:
“No neuron in natural or artificial isolation from other neurons has been shown capable of storing information in the usual notion of memory. … In particular, the possibility exists that extraneuronal compartments may participate importantly in the modulation of the wave process that characterize the intracellular records, and that these wave processes may rank at least equivalently with neuronal firing in the transaction of information and even more importantly in its deposition and recall.”
Finally, there were memory macromolecular notions advocated by Holger Hyden, based upon his finding of changes in the nucleotide composition of RNA. He proposed that learning gave rise to a specific pattern of instructional neural activity that altered the stability of RNA molecules, so that one base can exchange for another. In this way, new RNA molecules are formed with new base sequences that are specific to the instructing pattern of neural activity induced by learning. Hyden’s hypothesis thus implied that the patterns of stimulation activated by learning could introduce changes in RNA.
We were now therefore in a position to test experimentally which, if any, of these ideas had merit. Using the gill-withdrawal reflex, we quickly established that memory in the Aplysia nervous system is not represented in self-exciting loops of neurons but in changes in synaptic strength. We found that all three simple forms of learning – habituation, sensitization, and classical conditioning – lead to changes in the synaptic strength of specific sensory pathways, and that these changes parallel the time course of the memory process. These findings, which had been fully anticipated by our earlier studies of analogs of learning, gave rise to one of the major themes in our thinking about the molecular mechanisms of memory storage. Even though the anatomical connections between neurons develop according to a definite plan, the strength and effectiveness of those connections is not fully determined developmentally and can be altered by experience.
We therefore concluded the third of our 1970 series of consecutive papers in Science on the cellular mechanisms of learning with the following comments:
“… the data indicate that habituation and dishabituation (sensitization) both involve a change in the functional effectiveness of previously existing excitatory connections. Thus, at least in the simple cases, it seems unnecessary to explain the behavioral modifications by invoking electrical and chemical fields or a unique statistical distribution in a neural aggregate. The capability for behavioral modification seems to be built directly into the neural architecture of the behavioral reflex.
Finally, these studies strengthen the assumption … that a prerequisite for studying behavioral modification is the analysis of the wiring diagram underlying the behavior. We have, indeed, found that once the wiring diagram of the behavior is known, the analysis of its modification becomes greatly simplified. Thus, although this analysis pertains to only relatively simple and short-term behavioral modifications, a similar approach may perhaps also be applied to more complex as well as longer lasting learning processes.”
A beginning molecular analysis of memory storage
Having defined a critical site of plasticity, the situation became ripe for a molecular analysis. Here again I could not have been more fortunate. As I mentioned earlier, soon after I arrived at N.Y.U. I ran into James Schwartz. Jimmy had attended N.Y.U. Medical School two years behind me, but we had not really talked since I left N.Y.U. in 1956. After medical school Jimmy obtained a Ph.D. with Fritz Lipmann at the Rockefeller University, studying enzyme mechanisms and protein translation in cell-free bacteria extracts. As he and I began to talk again, he mentioned that he was thinking of moving from E. coli to the brain. Aplysia seemed ideal for biochemical study of individual nerve cells, so in 1966, Schwartz and I joined forces to carry out biochemical studies on individual identified nerve cells of Aplysia.
Jimmy soon showed that each nerve cell in Aplysia had a specific transmitter biochemistry. Cells that we had presumed on pharmacological grounds to be cholinergic did in fact synthesize and release acetylcholine. With time, Jimmy became interested in the molecular mechanisms of synaptic plasticity, and together we began to examine the role of protein synthesis in memory storage. We knew from the work of Louis Flexner and Bernard Agranoff in the mid 1960s that long-term memory in vertebrates required protein synthesis whereas short-term memory did not. In our first study together in 1971, we found that blocking protein synthesis for 24 hours did not prevent the short-term synaptic changes associated with habituation and sensitization. That finding made us think that short-term changes representing memory storage might involve activation of a second-messenger pathway, for example, the cyclic AMP (cAMP) cascade, whose actions might persist for periods longer than the millisecond duration of conventional synaptic actions.
In the discussion of our 1971 paper on the role of protein synthesis and synaptic plasticity, we wrote:
“Alterations in molecular configuration would not be expected to persist for long periods of time, although molecular changes lasting for several minutes have been observed. … Most likely, the biochemical mechanisms underlying these short-term plastic changes are composed of a series of sequential reactions which result in a new distribution of transmitter substance. Mechanisms involving cyclic 3′,5’AMP might serve as one example of a series of reactions which result in transient enhancement in the activity of a critical enzyme system. A pathway of this kind might trigger the mobilization of transmitter from one component (a long term store) to another (an immediately releasable store).
… If our conclusion is correct, … rapidly synthesized RNA cannot immediately play a role in neuronal functions; it might however, be important for long-term neuronal processes.”
Sutherland and Rall had already shown in brain slices that several neurotransmitters known to exist in the brain could increase the concentrations of cAMP by activating the enzyme adenylyl cyclase that converted ATP to cAMP. We appreciated that we had a particularly good experimental preparation for examining, on the cellular level, the role of second-messenger pathways in synaptic transmission, synaptic plasticity, and memory storage. In 1972, Schwartz, Howard Cedar, and I found that stimulation of the pathway involved in sensitization increased the level of cAMP in the entire abdominal ganglion. Schwartz and Cedar next found that the transmitter serotonin could also increase cAMP, providing the initial evidence that serotonin might activate an adenylyl cyclase in Aplysia.
Columbia University and the molecular analysis of short-term memory
It was at this time that I was invited to move from N.Y.U. to the Columbia University College of Physicians and Surgeons to become the founding director of the Center for Neurobiology and Behavior. I was able to persuade James Schwartz, Alden Spencer, and Irving Kupfermann (who was by then an Associate Professor, having established an independent research program concerned with feeding and motivational state in Aplysia) to join me. This move was attractive to me for several reasons. Historically, Columbia has had a strong tradition in neurology and psychiatry, and a friend and former clinical teacher, Lewis Rowland, was about to assume the chairmanship of the Department of Neurology. In addition, I had my first experience in neurobiology at Columbia with Harry Grundfest who was now retiring and I was being recruited to replace him. Finally, Denise was on the Columbia faculty and our house in Riverdale was near Columbia, thereby greatly simplifying our lives.
In 1974, just after arriving at Columbia, Castellucci and I went back to the elementary circuit of the gill-withdrawal reflex to determine the exact site of the synaptic change produced by short-term sensitization. We wanted to know which component of the synapse changes. Is it, as we suspected, based on indirect evidence, the presynaptic element of the synapse where chemical transmitter is released, or is it the postsynaptic site which contains the receptors which bind and respond to the transmitter? Using a quantal analysis, we found that the synaptic facilitation characteristic of sensitization is presynaptic and that inhibitors of serotonin block this presynaptic facilitation. Later, Hawkins and I found that tail stimuli that initiate sensitization activate a set of modulatory interneurons, the most important of which are serotonergic. The serotonergic and other modulatory interneurons all acted on the sensory neurons and on their presynaptic terminals to enhance transmitter release from their presynaptic terminals. We could now ask for the first time: Was cAMP directly involved in facilitation? In 1976, Marcello Brunelli could take advantage of the size of the Aplysia neurons and inject cAMP directly into the presynaptic sensory cell and thereby find a clear enhancement of synaptic transmission. This cAMP-induced enhancement paralleled the enhancement produced by serotonin or tail stimulation.
I now began to interact with Paul Greengard, who was demonstrating that cAMP produced its actions in the brain through the cAMP-dependent protein kinase, or PKA. In 1980, Schwartz, Castellucci, and I collaborated with Greengard. We injected a purified catalytic subunit of bovine PKA into presynaptic sensory neurons and found that it simulated the actions of cAMP or serotonin. Moreover, we could block the actions of serotonin by injecting into the sensory neuron the specific peptide inhibitor of PKA, protein kinase inhibitor PKI. With Steven Siegelbaum we next began to define some of the targets of PKA and focused on one target, a novel K+ channel. Steve showed that this channel is closed by serotonin and by PKA and that this closure is achieved in a manner consistent with the channel being phosphorylated directly by PKA.
The Howard Hughes Medical Institute and the molecular analysis of long-term memory
Just before I arrived at Columbia, Arnold Kriegstein, an M.D.-Ph.D. student, succeeded in culturing embryonic Aplysia in the N.Y.U. laboratory, a quest which had intrigued biologists and eluded their efforts for almost a century. Most of us who were there will not readily forget Kriegstein’s extraordinary in-house seminar in December, 1973 when he first described his discovery that the red seaweed Laurencia pacifica is required to trigger metamorphosis from a free-swimming veliger larva to a small crawling snail, a discovery that allowed him to show the first pictures of the beautiful tiny post-metamorphic juvenile Aplysia. I remember saying to myself. “Babies are always so beautiful!” Kriegstein’s work opened up the study of development and cell culture in Aplysia.
Because we now had young animals at all stages of development, we at last had the essential requirements for the generation of dissociated cell culture. This was taken on by Sam Schacher and Eric Proshansky. With the help of Steven Rayport (another M.D.-Ph.D. student at Columbia University), Schacher soon succeeded in culturing the individual sensory neurons, motor neurons, and serotonergic neurons of the gill-withdrawal reflex. The development of the culture system coincided with two other events that allowed me to begin studying the molecular mechanisms of long-term memory storage. The first was my encounter with Richard Axel and my collaboration in 1979, with him and with Richard Scheller, who became a joint post-doctoral fellow. The second was my being recruited to become a senior investigator at the Howard Hughes Medical Institute.
Axel and Scheller’s success in 1982 in cloning the gene encoding the egglaying hormone in Aplysia seeded Axel’s long-term interest in neurobiology and gave me not only a wonderful friend but also an exposure to the methods of recombinant DNA and modern molecular biology. The very next year, in 1983, Donald Fredrickson, the newly appointed President of the Howard Hughes Medical Research Institute, asked Schwartz, Axel, and me to form the nucleus of a Howard Hughes Medical Research Institute at Columbia devoted to molecular neural science. The Howard Hughes Medical Research Institute gave us the opportunity to recruit from Harvard both Tom Jessell and Gary Struhl, as well as to keep Steven Siegelbaum at Columbia.
My first goal on becoming a Highes Investigator was to examine the molecular mechanisms underlying the synaptic changes that parallel long-term memory storage. In 1885, Herman Ebbinghaus transformed speculation about memory into a laboratory science by having subjects memorize lists of nonsense syllables. In this way Ebbinghaus generated two basic principles about memory storage. First, he found that the transition from short-term memory to long-term memory is graded; practice makes perfect. Second, he anticipated the existence of a fundamental distinction between short- and long-term memory.
What, then, was the molecular basis for this fundamental distinction between short- and long-term memory? As we have seen, in the mid-1960s Flexner and Agranoff examined this distinction biochemically and found that inhibitors of protein synthesis disrupt long-term memory without adversely affecting learning, or short-term memory. We found that long-term sensitization in Aplysia is similarly dependent on protein synthesis, whereas short-term sensitization is not. These findings illustrated the generality of the distinction between short-term and long-term memory processes for both invertebrates and vertebrates. In each case spaced repetition of the learning stimulus acts to transform a transient memory into a more stable (long-term) form by means of a process that depends on new protein synthesis. But how this occurred was a mystery.
We had earlier found in Aplysia that long-term sensitization involved a persistent increase in the strength of the same synaptic connection altered by the shortterm process – the connections between the sensory and motor neurons of the gill-withdrawal reflex. To study this process more effectively we turned to dissociated cell culture and found that we could reconstitute both short- and long-term synaptic facilitation in a culture consisting of only a single sensory neuron and a single motor neuron. We did this together with Sam Schacher, Philip Goelet, and Pier Giorgio Montarolo by applying either one or five brief spaced pulses of serotonin to the sensory neuron and motor neuron in the culture dish. Much like behavioral long-term memory, the long-term synaptic changes required new protein synthesis while the short-term changes did not. Thus, we had trapped the protein synthesis-dependent component of memory storage in the elementary synaptic connection between two identified cells. We now could address directly the question: Why is protein synthesis required for long-term and not short-term facilitation? What are the molecular steps that switch on long-term facilitation and, once switched on, how is it maintained?
We next found that steps for new proteins are activated by a cascade of genes initiated by the cAMP-dependent protein kinase. With repeated application of serotonin, PKA translocates to the nucleus and in so doing activates the MAP kinase (mitogen activated protein kinase), another kinase often recruited for growth. Thus, one of the functions of repeated stimulation was to cause both kinases to move into the nucleus. Pramod Dash and Binyamin Hochner and later Cristina Alberini, Mirella Ghirardi, and Dusan Bartsch provided the first evidence that in the nucleus, these kinases act on a gene regulator called CREB-1 (the cAMP response element binding proteins) to initiate a cascade of gene actions. With David Glanzman and Craig Bailey, we found that the CREB-mediated gene cascade which triggers the synthesis of new protein is required for the growth of new synaptic connections and it is the formation of these new synapses that sustains the long-term change.
The requirement for transcription in long-term facilitation explained why long-term memory requires the synthesis of new proteins. However, this requirement now posed a cell-biological puzzle: if long-term synaptic change relies on the activation of genes in the nucleus, that means there is ready communication between the nucleus and the synapse. If that is so, must all such long-lasting changes in the signaling ability of the neuron be cell-wide? Or can long-term synaptic changes be restricted to individual synapses. Experiments by Kelsey Martin, based on a beautiful new cell culture system she developed, revealed that individual synapses or groups of synapses within a cell can be modified independently.
A return to the hippocampus: genetically modified mice and the study of complex spatial memory storage
In our studies in Aplysia we focused on the simplest forms of memory, called implicit (or procedural) memory. These memories are concerned with the unconscious recall of perceptual and motor skills and do not require a hippocampus. The hippocampus is involved in explicit (or declarative) memory, memory for people, objects, or places, memories that require conscious participation for recall. For years I tried to encourage people who left my lab to turn their attention to the hippocampus, but to no avail. Finally in 1990, when I reached my 60th birthday, I returned to the study of the hippocampus myself. I was emboldened to do so in great part because of the development of methods for inserting and for knocking out individual genes in mice. This work made it clear to me that mice offered a superb genetic system for examining the role of individual genes in synaptic modification on the one hand, and intact behavior – explicit memory storage – on the other. Mice have a well developed medial temporal lobe and hippocampus, and these are important for explicit memory of objects and space. Moreover, in 1972, Tim Bliss and Terje Lomo in Per Andersen’s laboratory in Oslo, had discovered that electrically stimulating any one of the three major pathways in the hippocampus gives rise to a synaptic facilitation, called long-term potentiation or LTP. We were interested in two questions: (1) What are the molecular signaling pathways that are important for LTP? (2) Is LTP important for explicit memory storage? In the move to genetically modified mice, the contributions of Seth Grant and Mark Mayford were particularly influential.
Grant was the driving force in our first studies, in which we showed a role for nonreceptor tyrosine kinases in long-term potentiation, and in spatial memory in the hippocampus. Mayford’s critical thinking became important somewhat later, as we began to realize the limitations in the first generation of genetically modified mice. The limitations stimulated Mayford to develop regionally restricted promoters that limited the expression of genes to only certain regions of the brain, and methods for controlling the timing of gene expression. Those two technical advances by Mayford proved important in allowing us, and Susumu Tonegawa (whose laboratory was now also focusing on studying memory in genetically modified mice), to generate mice whose phenotypes were more specific and in whom a genetic defect could be more readily interpreted than in the first generation of genetically modified mice because the defect could be related, somewhat more directly to specific synaptic changes and to behavior. Over the next few years Mayford, Ted Abel, Mark Barad, Isabelle Mansuy, Chris Pittenger, Amy Chen, and Angel Barco created a number of regionally restricted and regulated transgenic animals that allowed us to examine the role of the PKA- CREB-1 and CREB-2 and the protein synthesis-dependent transcriptional switch within the hippocampus, and to find that it was quite similar in principle to what we had encountered in Aplysia. Our lab and those of Alcino Silva and Dan Storm found that the cAMP, PKA, and CREB switch were required for long-term forms of synaptic plasticity in the hippocampus, was also required for spatial memory.
A molecular approach to the cognitive map of space in the hippocampus: steps toward a molecular biology of attention
With this background information about genes, LTP, and spatial memory, we now could ask a deeper question: How does an animal learn about extrapersonal space? Why does spatial memory go awry with defects in PKA signaling? What is the function of the transcriptional switch? To address these questions, we turned to studying how space is represented in the hippocampus.
One of the key insights to emerge from the study of higher cognitive functions is that each perceptual or motor act has an internal or neural representation in the brain. These representations can be either simple or complex. The simplest internal representations are those evident in the sensory systems where the afferent fibers are arranged as topographic maps of the receptor surface. These are the representations which Wade Marshall, my former mentor at the NIH, had discovered in the 1930s and early 1940s. Marshall showed that this map is most clearly evident in the neural representation of personal space, the representation of touch. The neural representation of the space surrounding the body, the extrapersonal space, is far more complex. Here the representation is not topographical but encoded in the pattern of firing of cells that do not have any specific topographic relation to one another with respect to the receptor surface. Thus, adjacent cells need not encode adjacent regions of extrapersonal space.
This representation was discovered in 1971, by John O’Keefe at University College London, who made the brilliant observation that the hippocampus has a cognitive map – a complete representation of extrapersonal space. O’Keefe discovered that all the pyramidal cells in the hippocampus, the very same cells that are used to study long-term potentiation have, as a natural function in the intact animal, the ability to encode space. He found that when an animal moves around in a familiar environment, different pyramidal cells in the hippocampus fire as the animal traverses different regions of the environment. This tendency is so marked that O’Keefe referred to the pyramidal cells as place cells. Some place cells may fire only when the animal’s head enters one position in a given space. Other pyramidal cells will fire when the animal’s head enters another position in the same space. Thus, a mouse’s brain breaks up the space in which it walks into many small overlapping fields, and each field is assigned to specific cells in the hippocampus, forming a spatial map of the animal’s surroundings. When the animal enters a new environment, a new place map is formed within minutes.
These observations have given rise to the idea that the hippocampus contains a map-like representation of the animal’s current extrapersonal environment, and that the firing of place cells in the hippocampus signals the animal’s moment-to-moment location within the environment. This spatial map is the best-understood example of a complex internal representation in the brain, a true cognitive map. It differs in several ways from the classical sensory maps found by Wade Marshall for touch, vision, or hearing. Unlike sensory maps, the map of space is not topographic, that is, neighboring cells in the hippocampus do not represent neighboring regions in the environment. Furthermore, a place cell will fire in the same place regardless of what the animal is looking at. Moreover, the firing of place cells can persist after pertinent sensory cues are removed and even in the dark. Thus, although the activity of a place cell can be modulated by sensory input, it is not determined by sensory input as is the case for the activity of neurons in a sensory system. It appears that the place cells do not map the current sensory input, but the location where the animal thinks it is in space.
Place fields are formed in minutes, and once formed the map to which they contribute can remain stable for weeks. It struck me in 1995 that formation of this internal representation – this cognitive map of space – was a learning process and that synaptic plasticity related to LTP might have a role in stabilizing this cognitive representation.
Although place cells have been studied since 1971, nothing was known about the cellular or molecular mechanisms whereby new place fields are formed, and specifically no one had attempted to relate the biology of place cells to the molecular mechanisms of LTP or hippocampal-based memory. To explore this problem, I was fortunate to start a collaboration with Robert Muller at Downstate Medical Center in Brooklyn, who had pioneered the systematic study of place cells. This problem was taken on by Cliff Kentros, a postdoctoral fellow in my lab, by Naveen Agnihotri, a graduate student, and by Alex Rotenberg, a joint student with Muller and myself. Using a combination of pharmacological and genetic approaches, we demonstrated a link between recruitment of PKA and protein synthesis on the one hand, and on the other, the long-term, but not short-term stability of the hippocampal representation of space. Thus, PKA and protein synthesis are required for longterm memories of extrapersonal space because that memory is based on a learned internal representation of space whose long-term stability requires PKA and new protein synthesis.
This raised a final question: Explicit memory in humans differs from implicit memory in requiring conscious attention for recall. How does conscious attention come to bear on explicit memory? Indeed, how can one study consciousness in the mouse? In the course of our work on place fields, Kentros, Agnihotri, Hawkins, and I found that the long-term stability of the place field map correlated strongly with the degree to which the animal was required to attend to its environment. This demonstrates that, rather than being an implicit, automatic, process, the long-term recall of a stably formed place cell map requires the mouse to attend to its environment, as would be expected for explicit memory in human beings. The finding that attention, the recruitment of PKA, and new protein synthesis are required to form and recall a stable map in the mouse has opened up a molecular biological approach to an attentional process.
From psychoanalysis to Aplysia to the role of attention in the cognitive representation of extrapersonal space
During the past 10 years my career has begun to come full circle. From an initial interest in the complex cognitive problems of psychoanalysis and memory storage, my research on memory led me first to the mammalian hippocampus, which proved too difficult as a first step and forced me to take a more reductionist approach and study initially the simplest forms of memory in Aplysia, and then, only much later, the more complex forms of memory in mice. I found that despite important differences in detail, simple implicit and explicit memories have a similar short- and long-term storage form. In each form, short-term storage requires covalent modification of pre-existing proteins leading to the alteration of pre-existing synaptic connections, whereas long-term memory storage requires gene activation, new protein synthesis, and the growth of new synaptic connections.
In the course of this work we began to explore how explicit memory storage for space affects the internal representation of space. We found that on the level of internal representation the storage mechanisms for explicit memory are similar to those in human beings in requiring attention. Attention is a component of conscious response, perhaps the great challenge of all research on mental processes. It thus seems likely that in future decades, the study of memory, perhaps even in mice, is likely to allow molecular insights into even the deepest problems of human behavior.
A personal perspective
Although doing research on Aplysia and the hippocampus and discussing science with colleagues in my lab have given me the greatest intellectual satisfactions, I have loved teaching and have learned a great deal from lecturing to medical and graduate students. It was in the context of the neural science course at Columbia that the idea arose of doing a textbook, Principles of Neural Science. In college and medical school I was never a good note-taker. I always preferred sitting back, enjoying the lecture, and just scribbling down a few words here and there. When I came to Columbia to develop the neural science course, I was struck by how much energy students were devoting to writing out every single word of lectures, and I wanted to help them get over that. I therefore encouraged the faculty to provide a syllabus for each lecture, and with time I edited the syllabus, added figures to it and improved it. Then Jimmy Schwartz and I decided that the syllabus was becoming sufficiently useful that we might make a textbook out of it. Our textbook was the first attempt to bridge cell and molecular biology to neural science and neural science to behavior and clinical states. The response to the first edition was so gratifying that we made an effort to make the book better and more complete. With the second edition, not only students but also scientists began to regard our textbook as useful. With the help of Tom Jessell, we further improved the third and fourth editions. The widespread reception of this book, both in the United States and abroad, has been a source of deep satisfaction to me and to the other contributors.
Outside of our work and our family, Denise and I enjoy the visual arts and classical music, especially opera. Our interest in both of these activities is greatly enriched by having within easy reach of our home the great museums and galleries of Manhattan as well as the Metropolitan Opera. We also are inveterate – I am tempted to say addicted – collectors of art and antiques. We have lived for 36 years in a now 150-year-old house in the Riverdale section of the Bronx, with wonderful views of the Hudson River and the Palisades. We collect French art nouveau furniture, vases, and lamps, an interest that originated with Denise and her mother, and graphic art of the Austrian and German Expressionists, an interest which originated with me. As I write this, I am beginning to suspect that our collecting may well be an attempt to recapture a part of our hopelessly lost youth.
In the course of my career I have incurred many debts both personal and scientific. First and foremost I owe an enormous personal debt to my parents and my brother Lewis. My parents were able in mid-life to relocate to a foreign country – my father spoke not a word of English when he first arrived in New York – and to create a new life for themselves and their sons. My parents not only succeeded in establishing themselves in their small store in Brooklyn, but were sufficiently successful to support me through college and medical school. They were so occupied with their store that throughout their life in America they did not share in the cultural life of New York, which Lewis and I were beginning to enjoy. Despite their constant labor they were always extremely optimistic and supportive of us, and never tried to dictate decisions about my work or play. Lewis was also an enormous influence on me in my early years, and my interest in classical music and my joy in learning were importantly influenced by him. While a graduate student at Brown University writing his dissertation in linguistics and Middle High German, he was called to service as an intelligence officer in the Korean War. He and his wife, Elise, went first in 1951 to Germany and then in 1953 to Paris, France, where he had a position as a civilian in Air Force Intelligence. He so enjoyed his life in France, that he lost his interest in an academic life and stayed in France for 13 years, where he and Elise raised five children. He eventually returned to the United States and finished his career in a series of administrative positions, in the Health Department of the City of New York. He died in 1979, at age 54 of a recurrence of a cancer of the kidney, which we all thought had been successfully removed when it first presented 10 years earlier.
Second, I have been privileged to enjoy a wonderfully supportive, endlessly interesting, and stable family life with Denise, my partner, best friend, and most honest critic for now 45 years. Throughout our life together she has consistently encouraged my love of research and supported my scientific aspirations. Denise is a professor in the Department of Psychiatry and in the School of Public Health at Columbia University, and has pioneered the study of drug abuse in adolescence. Her work on the epidemiology of drug abuse has become the basis of the current understanding of the developmental sequence whereby adolescents become involved in drugs. I am also greatly in debted to our two children, Paul and Minouche, for the joy they gave Denise and me while growing up and the satisfaction they have given us in seeing what principled and interesting people they have become and how thoughtful they are as parents to their own children. Our son Paul majored in economics at Haverford College and graduated from the Columbia Business School. He now manages a set of investment funds at Dreyfus-Mellon. Paul is married to Emily Kaplan, an interior designer; they live in Scarsdale, N.Y. and have two daughters, Allison (born on January 5, 1992) and Libby (born on October 14, 1995). Our daughter Minouche went to Yale College and Harvard Law School. She practices public interest law in San Francisco specializing in women’s rights and family violence. Minouche is married to Rick Sheinfield, also a public interest lawyer, and they have a son, Izak (born on November 10, 1998) and a daughter, Maya (born on March 12, 2001).
In retrospect it seems a very long way for me from Vienna to Stockholm. My timely departure from Vienna made for a remarkably fortunate life in the United States. The freedom that I have experienced in America and in its academic institutions made Stockholm possible for me, as it has for many others.
Postscripts: a Curriculum Vitae
I began my academic career at the Harvard Medical School, where from 1963 to 1965, I was an instructor in the Department of Psychiatry. In 1965, I moved to New York University as associate professor where, together with Alden Spencer and James Schwartz, we developed the first group in the country devoted to both cellular neurobiology and behavior. At the time I was recruited to N.Y.U., Denise was recruited to the Columbia University College of Physicians and Surgeons, where she gradually rose to the rank of professor.
In 1974, Harry Grundfest retired and I was recruited to Columbia to replace him. At Columbia I was the founding director of the Center for Neurobiology and Behavior. In 1983, I became a University Professor at Columbia. In 1984, I resigned as director of the Center to become a senior investigator at the newly formed Howard Hughes Medical Research Institute at Columbia.
Since 1974, I have been a member of the National Academy of Sciences USA. Later I became a member of the National Science Academies of Germany and France, the American Academy of Arts and Sciences, the American Philosophical Society, the National Institute of Medicine, and most recently, Germany’s Orden Pour Le Mérite für Wissenschaften und Künste. Being invited to join the Orden was for me a particularly great honor. The collection of scholars and scientists in the Orden is extraordinary; as an extra bonus it includes old friends such as the great German historian Fritz Stern, and a sterling group of biologists including Max Perutz, Christiane Nüsslein-Volhard, Bert Sakmann, Erwin Neher, Walter Gehring, Charles Weissman, and Robert Weinberg.
I have been awarded the Lester N. Hofheimer Prize for Research of the American Psychiatric Association (1977), the Karl Spencer Lashley Prize in Neurobiology from the American Philosophical Society (1981), the Dickson Prize in Biology and Medicine from the University of Pittsburgh (1982), the Albert Lasker Award (1983), the Rosenstiel Award of Brandeis University (1984), the Howard Crosby Warren Medal by the Society of Experimental Psychologists (1984), the American Association of Medical Colleges Award for Distinguished Research in the Biomedical Sciences (1985), the Gairdner International Award of Canada for Outstanding Achievement in Medical Science (1987), the National Medal of Science (1988), the J. Murray Luck Award for Scientific Reviewing from the National Academy of Sciences (1988), the American College of Physicians Award in Basic Science (1989), the Robert J. and Claire Pasarow Foundation Award in Neuroscience (1989), the Bristol-Myers Squibb Award for Distinguished Achievement in Neuroscience Research (1991), the Warren Triennial Prize from the Massachusetts General Hospital (1992), the Harvey Prize of the Technion in Haifa (1993), the Stevens Triennial Prize from Columbia University (1995), the Dana Award (1997), the Gerard Prize of the Society of Neuroscience (1997), the Wolf Prize of Israel (1999), and the Dr. A.H. Heineken Prize for Medicine from the Royal Netherlands Academy of Arts and Sciences in Amsterdam (2000).
I have received honorary degrees from nine universities, including three European universities: the University of Vienna, Edinburgh, and Turin. Surprisingly, the first honorary degree I received, in 1983, was from the Jewish Theological Seminary in New York. I was thrilled that they would even know of my work. I suspect they learned of that from my colleague Mortimer Ostow, one of the psychoanalysts who first stirred my interest in relating psychoanalysis and the brain. My father had already died but my mother came to the graduation ceremony, and in his introductory remarks Gerson D. Cohen, the chancellor of the seminary, referred to my having received a good Hebrew education at the Yeshiva of Flatbush, an acknowledgement which filled my mother’s Jewish heart with pride. As this recitation makes clear, I also owe a profound intellectual debt to my scientific teachers – Harry Grundfest, Dominick Purpura, Wade Marshall, and Ladislav Tauc – who tolerated my naivete and encouraged my brashness. I also benefited greatly from Steve Kuffler’s sage insight and advice and from Alden Spencer’s generous friendship. I also am indebted to the extraordinary collection of colleagues, fellows, and students that I have had the privilege of interacting and collaborating with and whose individual contributions I describe in more detail in my Nobel Lecture. Finally, I am deeply grateful to Columbia University and the Howard Hughes Medical Research Institute, two great institutions that have created open environments supportive of scholarship and research.
Selected References |
Adey, W.B. (1965) Electrophysiological patterns and cerebral impedance characteristics in orientering and discriminative behavior. Proc. Intern. Congr. Physiol. Soc. 23nd , Tokyo: 324-339. |
Berkley, G.E. (1988) Vienna and Its Jews. The Tragedy of Success, 1880-1980, with Forward by Harry Zoh. Abt Boos, Cambridge, Mass. |
Bukey, E.B. (2000) Hitler’s Austria. Popular Sentiment in the Nazi Era 1938-1945. The University of North Carolina Press, Chapel Hill, N.C. |
Burns, B.D. (1958) The Mammalian Celebral Cortex Monographs of the Physiological Society. Edward Arnold (Publishers) Ltd., London. |
Ebbinghaus, H. (1885) Memory: A Contribution to Experimental Psychology (1885). Reprinted, NewYork, Dover, 1963. |
Hebb, D. O. (1949) The Organization of Behavior, John Wiley & Sons, Inc., N.Y |
Hyden, H. and Egyhazi, E. (1962) Nuclear RNA changes in nerve cells during learning experiments in rats. Proc. Natl. Acad. Sci. USA 48:1366-1373. |
Kershaw, Ian (2000) Hitler 1936-1945 Nemesis, WM. Norton & Co., New York, NX |
Kuffler, SM. and Fzaguirre, C. (1955) Process of excitation in the dendrites and soma of single isolated sensory nerve cells of the lobsters and crayfish. J. Gen. Physiol. 39:87-119. |
Pulzer, Peter (1988) The Rise of Political Anti-Semitism in Germany and Austria. Harvard University Press, Cambridge, Mass. |
Ramón y Cajal, S. (1894) The Croonian Lecture: La Fine Structure des Centres Nerveux. Proc. R. Soc. London B., 56, 444-467. |
Schorske, Carl E. (1980) Fin de Siecle Vienna. Politics and Culture. Knopf, Inc., Random House, New York, N.Y. |
Weiss, D.W. (1999) Reluctant Return. A Survivor’s journey to an Austrian Town. Indiana University Press, Bloomington, Ind. |
Zuckmayer, C. (1966) A Part of Myself. Translated from the German by Richard and Clara Winston. Harcourt, Brace, Jovanovich, Inc., New York, N.Y. |
This autobiography/biography was written at the time of the award and later published in the book series Les Prix Nobel/ Nobel Lectures/The Nobel Prizes. The information is sometimes updated with an addendum submitted by the Laureate.
For more biographical information, see: Kandel, Erich R., In Search of Memory: The Emergence of a New Science of Mind. W.W. Norton, New York, 2006.
Nobel Prizes and laureates
Six prizes were awarded for achievements that have conferred the greatest benefit to humankind. The 12 laureates' work and discoveries range from proteins' structures and machine learning to fighting for a world free of nuclear weapons.
See them all presented here.