Thomas C. Südhof
Biographical
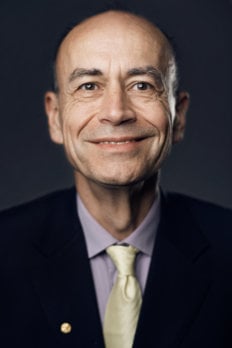
Upbringing
I was born in Göttingen on December 22, 1955. At that time, the aftermaths of the Second World War were still reverberating. Mine was an anthroposophical family; my maternal grandparents had been early followers of Rudolf Steiner’s teaching, and worked for Waldorf schools when Hitler assumed power and banned the anthrophosophical movement. Waldorf schools were forced to close, and my grandfather was conscripted to work in a chemical munitions factory – it was a miracle he survived the war. My uncle was drafted into the army out of school, and when I was born, he had just returned from the Soviet Union after 10 years as a prisoner of war. I was the second of four children. My parents were physicians, with my father pursuing a career in academic medicine, while my mother cared for our growing family. My father’s training led him to the United States during the time I was born; as a result, he learned of my arrival by telegram as he was learning biochemical methods in San Francisco, close to where – by a twist of fate – I now live.
I spent my childhood in Göttingen and Hannover, and graduated from the Hannover Waldorf School – resurrected after the war – in 1975. My strongest childhood memories were those of my maternal grandmother telling me stories about the time during the war, how she was reading Dostoyevski while trying to escape the bombs in underground shelters and hoping that my grandfather would survive. She imbued me with the importance of Goethe and detested Kant, whom I learned to love. I learned from my grandmother how important an intellectual life is under any circumstance, and that values are spiritual even if you are an atheist.
My father was a successful doctor who managed an entire hospital district and wrote countless books on general internal medicine; he worked very hard, and was continuously frustrated by what he felt were the inadequacies of the medical care system and the academic world. However, when I was in high school, my father died of a heart attack, brought about by inattention to his health, and my mother had to cope with life alone with four children – a difficult and sad but an also partially liberating experience for her, as she explained to me later. Her strength was an example to me, her ability to accept what happened without giving up, and to concentrate on what was important to her.
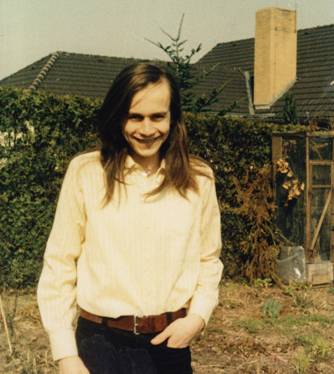
I had been interested in many different subjects in high school, in fact all subjects except for sports which I found primitive – now ironic to me as I have become addicted to regular exercise. Early on, I became fascinated by classical music. After unsuccessful attempts at playing the violin, I gave this instrument up to the delight of everyone around me who had to listen to me trying. However, I then decided to learn to play bassoon, which I pursued with a vengeance, motivated by a wonderful teacher (Herbert Tauscher) who was the solo-bassoonist at the local opera house, and who probably taught me more about life than most of my other teachers. I credit my musical education with my dual appreciation for discipline and hard work on the one hand, and for creativity on the other. I think trying to be marginally successful in learning how to be a musician taught me how to be a scientist: there is no creativity if one does not master the subject and pay exquisite attention to the details, but there is also no creativity if one cannot transcend the details and the common interpretation of such details, and use one’s mastery of the subject like an instrument to develop new ideas.
I did not know what to do with my life after school, except that I was determined not to serve in the military. More by default than by vocation, I decided to enter medical school, which kept all avenues open for a possible career in science or as a practitioner of something useful – as a physician – and allowed me to defer my military service. I thought that music, philosophy, or history were more interesting subjects than medicine, but I did not feel confident that I had sufficient talent to succeed in these difficult areas, whereas I thought that almost anybody can become a reasonably good medical doctor.
First Experiments
I studied first in Aachen, the beautiful former capital of Charles the Great, and then transferred to Göttingen, the former scientific center of the Weimar republic, in order to have better access to laboratory training since I became more and more interested in science.
Soon after arriving in Göttingen, I decided to join the Dept. of Neurochemistry of Prof. Victor P. Whittaker at the Max-Planck-Institut für biophysikalische Chemie as a ‘Hilfswissenschaftler’ (literally an ‘assisting scientist’, but more accurately a kind of ‘sub-scientist’). I was attracted to Whittaker’s department because it focused on biochemical approaches to probe the function of the brain, following up on Whittaker’s development of purification methods for synaptosomes and synaptic vesicles in the two preceding decades. Moreover, when I entered his lab, Whittaker had become increasingly interested in the cell biology of synaptic vesicle exo- and endocytosis, which I thought were fascinating. However, I never got a chance to work on the brain or synaptic vesicles when I was in Whittaker’s lab. As a lowly ‘Hilfswissenschaftler’, I was assigned to the task of examining the biophysical structure of chromaffin granules, which are the secretory vesicles of the adrenal medulla that store catecholamines. Although my project developed well, I started exploring other questions in parallel as I became more and more familiar with doing experiments, while simultaneously studying medicine at the university. A helpful factor was that my supposed supervisor, a senior US scientist who worked with Whittaker, departed soon after I started in Whittaker’s lab, leaving me completely alone in my experiments since Whittaker was not really interested in that work. I am infinitely grateful to Victor Whittaker for giving me complete freedom in his department in pursuing whatever I thought was interesting. I continued working in his department after my graduation from medical school in 1982 until I moved to the US a year later in 1983.
Among the work I performed during my time in Whittaker’s department in Göttingen, the most significant is probably the isolation and characterization of a new family of calcium-binding proteins that we called ‘calelectrins’ because we had initially purified them from the electric organ of Torpedo marmorata, although we also identified them in bovine liver and brain. ‘Calelectrins’ were among the first identified members of an enigmatic and evolutionarily ancient family of calcium-binding proteins now called annexins. Annexins were at the same time discovered in several other laboratories, and I am proud of the fact that we contributed to the first description of this intriguing protein family, although to this date their function remains unknown.
Postdoctoral Training
After finishing medical school, I decided to become an academic physician, along the mold of my deceased father. Although my time in Whittaker’s laboratory had taught me to love doing science, I wanted to do something more practical, exciting, and immediately useful than what I had seen in the Max-Planck-Institut in Göttingen. The standard career for an academic physician in Germany was to go abroad for a couple of years to acquire more clinically oriented scientific training before starting her/his clinical specialty training. Upon surveying the scientific landscape, I decided to join the laboratory of Mike Brown and Joe Goldstein at the University of Texas Southwestern Medical School in Dallas for postdoctoral training. Brown and Goldstein were already famous for their brilliant cell-biological studies when I made this decision. They were equally renowned for using cutting-edge scientific tools to address a central question in medicine, namely how cholesterol in blood is regulated. When I announced my decision to go to Dallas instead of the more conventional Boston or San Francisco, my friends and family were disappointed, but it was the best professional decision I ever made.
While in Joe Goldstein’s and Mike Brown’s laboratory, I cloned the gene encoding the LDL receptor, which taught me molecular biology, revealed to me the beauty of sequences and protein organizations, and opened up genetic analyses of this gene in human patients suffering from atherosclerosis. I also became interested in how expression of the LDL receptor is regulated by cholesterol, and identified a sequence element in the LDL receptor gene called ‘SRE’ for sterol-regulatory element that mediates the regulation of the LDL receptor expression by cholesterol. During my time in their laboratory, Joe Goldstein and Mike Brown were awarded the Nobel Prize (in 1985), which I still consider one of the best Nobel Prizes given. After I left, discovery of the SRE led to the identification of the SRE-binding protein in Brown and Goldstein’s laboratory, which in turn identified new mechanisms of transcriptional regulation effected by intramembrane proteolysis.
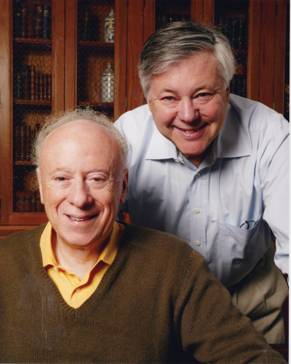
The contrast between Göttingen and Dallas could not have been bigger. When I arrived in Dallas, Texas was not yet the extremely conservative bastion of religious fundamentalism that it is now, but a vast state with an optimistic ‘can-do’ culture that was very different from the culture to which I had been exposed in Göttingen. The difference in scientific environment was even more extreme. In Dallas, scientific life was teeming with enthusiasm and energy, work was a pleasure, and excitement was pulsating through every experiment because the importance of the goals was self-evident. In Göttingen, as I realized when I was in Dallas, although the approach was very scholarly in the sense of pursuing knowledge, much of that pursuit was without regard to the importance of the subject. As a result, people often asked uninteresting questions, and possibly even wasted their time. To this date, I find it one of the hardest challenges in science to achieve the right balance between trusting my own judgment and listening to others. If I only rely on my own judgment, there is no corrective for mistakes, no adjustment of unreasonable impressions. However, if I listen only to the ‘world’, I will only follow fashions, will always be behind, and often will be just as wrong. Among the many things I credit Brown and Goldstein with for teaching me, the realization of this challenge and their example of how to deal with this challenge is among the most important. This challenge resembles that of composing music in which pure harmony is boring and meaningless, but pure dissonance is unbearable, and it is really the back and forth between these extremes that creates meaning.
Early Years of the Südhof Lab
In 1986, at the end of my postdoctoral training, I faced the choice of resuming my clinical training, or of establishing my own laboratory. Probably the best advice Brown and Goldstein gave me was now: they suggested I forego further clinical training and do ‘only’ science, and they backed up this advice by providing me with the opportunity to start my own laboratory at Dallas. This I did, and ended up staying for another 22 years, interrupted only by a short guest appearance as a Max-Planck Director in Göttingen (see below).
When I started my laboratory at Dallas in 1986, I decided to attack a question that was raised by Whittaker’s work, but neglected since: what are synaptic vesicles composed of, and how do they undergo exo- and endocytosis, i.e., what is the mechanism of neurotransmitter release that underlies all synaptic transmission? We had learned from Whittaker’s work that synaptic vesicles could be biochemically purified, but nothing was known about the molecular mechanisms guiding synaptic vesicle exo- and endocytosis. Our initial approach, performed in close collaboration with Reinhard Jahn, whose laboratory at that time had just been set up in Munich, was simple: We set out to purify and clone every protein that might conceivably be involved, and worry about their functions later. This approach was initially criticized for being too descriptive, but turned out to be more fruitful than I could have hoped for, and has arguably led to a plausible understanding of neurotransmitter release.
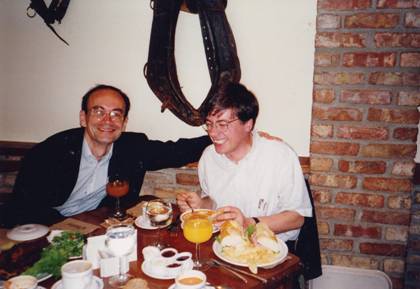
In the nearly three decades since I started my laboratory, our work, together with that of others, led to the identification of the key proteins that are involved in synaptic vesicle exocytosis. In particular, this work shed light on the molecular mechanisms underlying membrane fusion during synaptic vesicle exocytosis, explained how calcium signals control these mechanisms, and described the molecular organization of the presynaptic terminal that allows fast coupling of an action potential and the ensuing calcium influx to neurotransmitter release. Some of the proteins whose function we identified are now scientific household names and have general roles in eukaryotic membrane fusion that go beyond a synaptic function, while other proteins are specific to synapses and in part account for the exquisite precision and plasticity of synapses as elementary computational elements in brain. I feel fortunate to have stumbled onto this overarching neuroscience question at a time when it was ready to be addressed, and it has been tremendous fun to work our way through the various synaptic proteins and their properties that shape the functions of these proteins.
It is important to note, however, that the nature of our studies was not revolutionary. In my career, no single major discovery changed the field all at once. Instead, our work progressed in incremental steps over two decades. I think this is a general property of scientific progress in understanding how something works – a single experiment rarely explains a major question, but usually a body of work is required. In contrast, scientific progress in developing tools normally advances in spurts, and often a single flash of genius creates a completely new method (e.g., see monoclonal antibodies, patch clamping, PCR, or shRNAs, to name a few).
The closest our work came to inducing a radical change in the field was probably the identification of synaptotagmins as calcium-sensors for fusion, and of Sec1/Munc18-like proteins (SM-proteins) as membrane fusion proteins, but both hypotheses took decades to develop and to become accepted by the field – in fact, the SM-protein hypothesis was only recently adopted by others, 20 years after we proposed it, and is still in flux. Thus, our work in parallel with that of others (Reinhard Jahn, James Rothman, Jose Rizo, Randy Schekman, Richard Scheller, Cesare Montecucco, and Axel Brunger come to mind) produced a steady incremental advance that resulted a better understanding of how membranes fuse, one step at a time. As a result of this combined effort, we now know that SNAREs are the fusion catalysts at the synapse, first shown when SNAREs were shown to be the substrates of clostridial neurotoxins, that SM-proteins in general and Munc18-1 in particular are essential contributors to all membrane fusion events, that a synaptotagmin-based mechanism assisted by complexin underlies nearly all regulated exocytosis, and that synaptic exocytosis is organized in time and space by an active zone protein scaffold containing RIM and Munc13 proteins as central elements.
![The Südhof laboratory in Dallas in 1995. Sitting in the first row left to right: Thomas Rosahl, Martin Geppert, Ewa Borowicz, Izabella Kornblum [sitting behind the row], Else Fykse, Cai Li, Andrea Roth, Shirley Clement, Christopher Newton, and Greg Mignery. Standing left to right: Konstantin Ichtechenko, Alexander Petrenko, Thomas Südhof, Beate Ullrich, Andrei Khokhlatchev, Yutaka Hata, and Harvey McMahon.](https://www.nobelprize.org/uploads/2018/06/sudhof-bio-4-lab.jpg)
The work in my lab would have been impossible without the contributions of many brilliant postdoctoral fellows who have now gone on to successful careers on their own. Ever since I started my laboratory, I have found the pleasure of working with others the best part of my life. The continuing friendship of my former trainees has been one of the major satisfactions of my career. Among these were Mark Perin with whom I cloned synaptotagmin, Yutaka Hata who discovered Munc18, Martin Geppert who performed the initial mouse genetics experiments in my lab, Nils Brose who identified Munc13, Harvey McMahon who identified complexins, Yun Wang who isolated RIM, and many others who made essential contributions. Complementing these great co-workers, I had the best collaborators I could possibly wish for. Besides Reinhard Jahn (who had moved to Yale after Munich, and then on to Göttingen), the most important of these collaborators were Jose Rizo in Dallas with whom we worked out the atomic structures of many of the proteins we studied, Bob Hammer in Dallas who helped us with the mouse genetics, and Chuck Stevens at the Salk Institute who introduced us to the beauty of electrophysiological analyses.
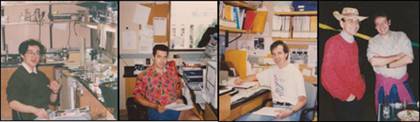
My German Intermezzo
Ten years after I started my laboratory, while the work described above was progressing, I was offered the opportunity to return to Germany and to organize a Department of Neuroscience at the Max-Planck-Institut für experimentelle Medizin in Göttingen, my home town. I enthusiastically took on the challenge, planned and oversaw the building of a new animal facility, hired scientists, and organized the renovations and equipment of a suite of laboratories. However, after a few years the leadership of the Max-Planck-Society changed. It soon became clear that the Max-Planck-Society’s new president, Prof. Hubert Markl, developed doubts about my recruitment, and wanted to rebuild the institute that I was recruited into in directions that were quite different from what I had been promised. In a personal discussion, Prof. Markl suggested I resign my position at the Max-Planck-Institut and look for a future in the U.S., which I did.
I have never regretted my work for the Max-Planck-Institut in Göttingen, which laid the foundation for much of what happened there subsequently, including the subsequent recruitment of one of my postdoctoral fellows (Nils Brose) as a new director who has done a much better job than I could have done. However, I have also never regretted following Prof. Markl’s suggestion and returning to the U.S., where the breadth and tolerance of the system allowed me to operate in a manner that was more suitable for my somewhat iconoclastic temperament. Overall, my work as a director at the Max-Planck-Institut in Göttingen was a very positive experience that shaped my thinking when I subsequently had the opportunity to help build the Department of Neuroscience at the University of Texas Southwestern Medical Center in Dallas.
Maturity
Soon after I returned full-time to UT Southwestern at Dallas in 1998, I accepted the position of director of the Center for Basic Neuroscience, which was later transformed into the Department of Neuroscience. Building a Center and Department of Neuroscience partly occupied the following ten years, and was a lot of fun. Southwestern had a free-flowing and unbureaucratic environment that was extremely supportive. It was a pleasure to hire young people and see them develop, and I greatly appreciated the support of my colleagues in every respect.
Scientifically the 10 years between 1998 and 2008 were even more important. The flurry of discoveries of the 1990s created the impression that everything was already solved in membrane fusion and neurotransmitter release, but nothing could have been farther from the truth. I decided to continue to work on these questions, and believe that some of the most important observations in
the field came out of our work during that time period.
For example, it was well established in 2000 that SNAREs ‘do’ fusion and that they ‘do so’ by pulling membranes together, but it was unknown whether SNAREs were just nanomachines that acted as force-generators in approximating membranes, or whether they actually catalyze the fusion process, possibly by their transmembrane regions. Similarly, although we found that the SM-protein Munc18-1 was absolutely essential for fusion, Munc 18-1 appeared to bind to a form of the SNARE protein syntaxin-1 that was ‘closed’ and was thought to block fusion, whereas the yeast homolog Sec1p, as shown in elegant work by Peter Novick, bound to assembled SNARE complexes. How could the same protein be essential for fusion and inhibit SNARE-complex formation? Comparably puzzling questions surrounded the role of synaptotagmin as a calcium sensor in neurotransmitter release. Furthermore, a major question in understanding synapses had never been addressed, namely how the presynaptic machinery is organized in a manner that allows tight coupling of calcium-influx to the calcium-triggering of release. The significance of this latter question is often underestimated outside of the esoteric realm of neurophysiologists, but this tight coupling is the most important prerequisite for the speed and brevity of neurotransmitter release – in essence this coupling is what makes a synapse precise.
In the years after 1998, my lab and the labs of others, foremost those of my former postdoctoral fellows Nils Brose, Harvey McMahon, and Matthijs Verhage, of Christian Rosenmund, of Peter Novick, and of Jim Rothman, established several key points that address these questions. The most important was the demonstration that synaptotagmin is truly the calcium-sensor for release by showing that point mutations in synaptotagmin that change its calcium-affinity change release accordingly. Maybe equally important was the finding that Munc18 acts by binding to SNARE complexes after assembly, not by binding to one SNARE protein before assembly. Other significant findings of these years included the demonstration of the priming function of Munc13, the discovery that complexin acts as a ‘sidekick’ to support synaptotagmin function and that both synaptotagmin and complexin clamp minis in addition to the major action as activators of release, and that multiple synaptotagmins generally function as calcium-sensors in release. Moreover, in these years we identified specific chaperones that support the proper folding of SNAREs, opening up a new perspective on how SNARE function is maintained in neurons, an important issue because the loss of these chaperone activities were found to cause neurodegeneration. These were very productive years that did more than complete the stories we had begun in the 90s – they extended these stories into new directions, including an explanation of at least some forms of neurodegeneration. The one major issue that remained unresolved was how calcium-channels are recruited to the active zone, a question that was really only resolved after I moved to Stanford in 2008.
New and Old Directions
The currently final chapter in my career began when I moved my laboratory from UT Southwestern to Stanford University in 2008. After 10 years as a chair of a Neuroscience Center and then Department in Dallas, I felt that I wanted to devote more of my time to pure science, and to embark on a new professional direction, with an environment that was focused on academics. Moreover, I decided to redirect a large part of my efforts towards a major problem in neuroscience that appeared to be unexplored: how synapses are formed. Thus, in this currently last chapter of my work, I am probing the mechanisms that allow circuits to form in brain, and to form with often nearly magical properties dictated by the specific features of particular synapses at highly specific positions. I am fascinated by the complexity of this process, which far surpasses the numerical size of the genome, and interested in how disturbances in this process contribute to neuropsychiatric diseases such as autism and schizophrenia. This is what I would like to address in the next few years, hoping for at least some interesting insights.
As early as 1992, my laboratory had identified a family of cell-surface proteins called neurexins whose properties suggested that they may be involved in synapse formation. Neurexins were discovered because they are presynaptic receptors for the black widow spider venom component α-latrotoxin which paralyses small prey by causing excessive neurotransmitter release. However, the importance of neurexins and their ligands – such as the neuroligins, cerebellins, and neurexophilins which we and others identified – only became apparent in recent years when we started to analyze mouse mutants of these proteins.
Apart from these new directions, at Stanford we followed up on two ‘old’ questions about release: how calcium-channels are recruited to active zones, and what mediates the calcium-triggered release that remains in synapses which lack fast synaptotagmin calcium-sensor isoforms. Both questions had haunted me for decades – I was convinced of their importance but could not solve them. Only in the last few years did we develop answers to these questions in identifying the scaffolding proteins RIMs and RIM-BPs as the organizers of calcium-channels in the presynaptic active zone, and another synaptotagmin isoform (synaptotagmin-7) as a calcium-sensor for the remaining release in synaptotag-min-1 deficient forebrain neurons. Fittingly, this last observation was submitted a few weeks before the announcement of the Nobel Prize, and published coincidently with the award ceremony!
Life Lessons
After nearly 60 years of life and nearly 40 years as a scientist, I would like to draw the following personal conclusions, none of them very original. First, being a scientist, although socially a privilege and luxury, is not socially rewarding – for personal happiness, this profession is only worth it if a person obtains individual satisfaction in doing science. A scientist has to have the attitude of an adherent to Philip Spener’s pietism in Lutheran Germany in the 18th century – what counts are not outward successes, money, and social decorations, but the conviction of truth obtained from personal inspection of the evidence. After a glorious period of ascendance in Western Europe from Bacon’s England over Courvoisier’s France to Boltzmann’s Germany, the scientific method is now increasingly being challenged based on ideological grounds. In the most powerful country of the world, the United States, the majority of the governing elite at present feels free to dismiss some established scientific facts as fantasy, even suspecting evolution or climate science as communist conspiracies at a time when there is no communism left anywhere. At this stage – different from previous centuries – the only reason to pursue a career in science is an enormous curiosity to know what is really true.
Second, I at least have learned most from personal contacts, not from reading the literature or listening to talks. Although reading books or papers provided me with an indispensable background of facts, I learned how to think, how to assess a subject, and how to value a perspective from insightful comments of others. Thus, for a scientific career the most important elements are good teachers and mentors, and a great environment – not only during early years as student and postdoc, but throughout the entire career of a scientist. Now at an arguably rather advanced stage of my career, I need mentors and teachers more than ever – I need people who know better than I to tell me when I am wrong, and to make me aware of my mistakes! As an immediate consequence of this realization, I would advise everybody to make career choices primarily based on the people involved, not on the geographic location of a place or the fashionableness of the subject or the techniques. I believe this is true for all stages of a career.
Third, once a scientist has the opportunity to choose what to work on (increasingly a rarity in our world where political prescriptions of what scientists are supposed to discover are becoming more and more prevalent), he/she should make sure that whatever the choice of subject is, it is both important and tractable. I am personally often amazed about the choice of subject by some of my colleagues, possibly because I simply fail to recognize the importance of the subject. However, if one looks at the history of science over the last 50 years or so, I think one can argue that some approaches and goals have proved to be highly productive whereas others have not. For example, investments into bacterial and bacteriophage genetics early on eventually led to the golden age of molecular biology that all of science, but particularly cellular neuroscience, has benefitted from, whereas the parallel large investments into systems neuroscience has only recently started to bear some fruit after the tools developed by molecular and cellular neurobiology were beginning to be applied.
Finally, quantity doesn’t matter very much, nor does the place one publishes – in the end what counts is discovery which is often not immediately apparent. Most articles in “high-impact journals,” although highly cited initially, are soon forgotten. Especially in our time, when it is basically the editor of a journal and not the reviewers who decides what gets published – an editor who often has limited knowledge of the subject but knows what is ‘exciting’ – the major journals publish many papers that are composed of true data with contrived interpretations and very little long-term import. As scientists, we have to intellectually dissociate ourselves from fashion and journals, and focus on what is the actual content of a study, what the data really say (not what the abstract says!). We can then take pride and pleasure in work that reports an actual advance – I feel that this is the most important ability I tried to learn from my mentors, and I am trying to teach my students.
Thank You
Throughout my career, I have been generously supported by the Howard Hughes Medical Institute and the National Institute of Mental Health. I am grateful to both for their unflinching support. I have received several recognitions, all of them unexpected, among which I particularly cherish the Alden Spencer Award from Columbia University in 1993, the von Euler Lectureship from the Karolinska Institutet in 2004, the Kavli Award in 2010, the Lasker~deBakey Award in 2013, and – of course – the Nobel Prize. I am not sure I deserve any of these awards, as conceptual advances in science always represent incremental progress to which many minds contribute, but I immensely appreciate receiving them. Finally, I feel indebted beyond words to my family, my wife Lu Chen and my children Saskia, Alexander, Leanna, Sören, Roland, and Moritz, without whom I would be barren and rudderless, and who have been more considerate of me than I deserve, and finally to my ex-wife Annette Südhof who greatly supported me in earlier stages of my life, and to my brothers Markus and Donat Südhof and my sister Gudrun Südhof-Müller whom I appreciate more the older I get.
This autobiography/biography was written at the time of the award and later published in the book series Les Prix Nobel/ Nobel Lectures/The Nobel Prizes. The information is sometimes updated with an addendum submitted by the Laureate.
Nobel Prizes and laureates
See them all presented here.