John B. Fenn
Biographical
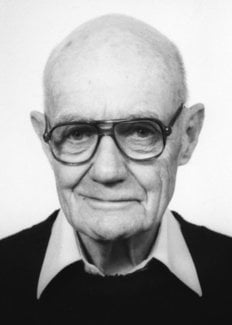
My father, Herbert Bennett Fenn, the eldest of three children was born and raised on a farm in northern Delaware which his father operated but did not own. I never saw that farm but I vividly remember my Grandmother’s frequent reference to a single chestnut tree in the front yard, so large and prolific that the nuts from that one tree paid the taxes on the farm every year! My mother was the sixth of ten children in the family of John Clarence Dingman, a country doctor in Spring Valley, N.Y. whose three surviving sons also became physicians. Dad worked his way through college, graduating in Electrical Engineering from Rutgers in 1910, the same year that mother received a degree in Home Economics and Nutrition from Columbia. They were both hired by the Presbyterian Mission Board to teach at the Sheldon Jackson Mission School in Sitka, originally settled by the Russians and sometime capital (until replaced by Juneau in 1900). The Chairman of that Board told each one about the other and warned them both not to fall in love, but if they did and got married he would provide them with a house for as long as they stayed in Sitka! They did and he did, so after mother’s death in 1990 (Dad had died in 1944) we found in her papers a clipping from the Sitka paper with a photograph of the “Honeymoon Cottage”.
During a cruise in 1996 I spent a day in Sitka. The Mission School is now Sheldon-Jackson University. I visited the library, discovered a complete file of student newspapers, and found several articles about my parents! Moreover, the present Librarian was then living in the “Honeymoon Cottage” and gave me a tour so I was actually able to look out through its front windows and see the magnificent view of Sitka Bay that my parents used to rave about.
The newlyweds were very happy in Alaska and no doubt would have remained there indefinitely had it not emerged that mother would be unable to bear children except by Caesarian Section, a procedure not then available in Sitka. Determined to have a family she persuaded Dad to move back to the States where he became manager of the Metacloth Company, a small enterprise in Lodi, N.J. whose main product was cotton duck treated by immersion in a concentrated ammoniacal solution of copper hydroxide followed by a wash in acid. The deep blue “cuprammonium” solution dissolved some of the cellulose which reprecipitated during passage through the succeeding acid bath, filling the pores of the cloth enough to make it “water resistant” but not “water-proof”. Residual copper provided a characteristic blue-green color along with a high resistance to attack by micro-organisms and white ants. For these reasons, “Metacloth” was in fairly steady demand for tents and tarpaulins in tropical latitudes. My abrupt introduction to “chemistry” occurred on one of the treasured Saturdays when Dad took me to the plant. Nosing around outside I lifted a small flap on the cover of a large tank half full of cuprammonium solution. I still recoil at the memory of that ammoniacal, and demoniacal, assault on my eyes and nose. It was a startling revelation on how and why a whiff of smelling salts can often revive people from a dead faint!
Our home was in Hackensack, N.J., next door to Lodi and County Seat of Bergen County. I was born in New York City in 1917 and three plus years later my brother Norman arrived in Paterson, N.J. where two of mother’s brothers were surgeons. The Metacloth company was sold in 1926 and Dad was unceremoniously dumped by the new owners. He was not a vindictive man but he got no little satisfaction out of the several occasions in the next two years when his help was needed in overcoming mistakes made by the new management. Meanwhile, approaching fifty and finding equivalent jobs scarce, he was paying the bills by working as a draftsman at the Fokker Aircraft Company in Teterboro, N.J. The value of having a trade as back-up for a profession was an enduring object lesson for a youngster on the eve of the Great Depression but there was for him a far more exciting consequence of the Fokker connection. When Lindbergh’s “Spirit of St. Louis” was shipped back from Paris after its famous flight across the Atlantic, it was parked for a time in a hangar at the Teterboro airport. The thrill of a lifetime for a ten year old boy was when his Dad took him into that hangar where he was allowed to sit in the cockpit and move the controls, pretending to be pilot of that famous plane!
Meanwhile the family fortunes were going down hill, much further than my brother and I were aware. Just before Dad lost his job, he and mother had invested their life savings in a new (for us) house that everyone insisted was worth “every nickel” of the $15,000 that it cost! Unfortunately, after we had to move and before the house could be sold the great depression had begun. No prospective buyers had enough nickels so those savings disappeared in the meltdown of foreclosure. Meanwhile, a door of opportunity had opened in Berea, Kentucky, a small community of 3500 or so inhabitants at the edge of bluegrass country some 40 miles south of Lexington and roughly halfway between Cincinnati, Ohio and Knoxville, Tennessee. The town of Berea was home for a remarkable institution known by the same name but officially entitled “Berea College and Allied Schools”. Both the community and the school had their roots in a non-sectarian Union Church founded in 1848 by John G. Fee, on a ridge of land donated by Cassius Clay, a brother of Henry Clay, the famous orator and statesman from Lexington, Ky.
Fee was a Congregational Minister from Massachusetts and a fervent abolitionist determined to provide educational opportunities for needy students, regardless of their race or creed. After decades of effort by himself and his followers his dream grew into what in 1928 comprised a coeducational student body of about 1700 divided among four schools: (1) The Foundation-Junior-High School with an ungraded program into which students with as little as two years of formal schooling could enroll and progress at their own rate up through the equivalent of 8th grade into a standard and accredited 9th grade curriculum; (2) The Academy, with an accredited curriculum for grades 10 through 12, (3) the Normal School with a two year program leading to a Teaching Certificate, and (4) the College which offered accredited curricula leading to BA degrees in the liberal arts and sciences as well as BS degrees in Home Economics and Agriculture. Mother’s sister was teaching in the College and knew that the Industrial Arts Department of the Foundation School and the Academy needed someone to teach Auto Mechanics and Practical Electricity. Dad was eminently qualified and got the job. We moved to Kentucky in time for me to enter the eighth grade in the “Training School” of Berea’s Normal School in the fall of 1928. The next fall I entered ninth grade in the Foundation School and continued on through the Academy and College.
A lot of misgivings, pain and confusion attended our passage through the newly opened door, including an automobile accident on the way to Kentucky, but life on the other side turned out to be rich and rewarding beyond what any of us had dreamed. In later years Dad and Mother both said, time and again, that losing his “good” job in New Jersey turned out to be the greatest blessing that we could have received! To this day my brother and I share those sentiments and count ourselves especially privileged to have been reared in what was a truly remarkable community. Its soul was its President, William J. Hutchins, father of Robert Maynard Hutchins, the “boy wonder” of the American education scene who became Secretary of Yale at the age of 24, Dean of its Law School at 26 and President of the University of Chicago at 30! “William J”, as the father was fondly known at his own institution, was truly one of nature’s noblemen. A striking man of vision and patrician to the core, his Berea was a singular stage on which the play was always provocative and the message meaningful. Name an outstanding man or woman of letters, the arts, science, or religion, of those days and the odds are high that he or she came to Berea to talk at one of the thrice weekly “United Chapels” for students from all schools. Attendance was required of all, and resented by most, but at my 50th class reunion there was a remarkable consensus among my surviving classmates that the community experiences of those United Chapel services were by far the most memorable and valuable components of their educational. Alas, that tradition has long since disappeared, one more victim of “students’ rights” and television. Moreover, as the public education system improved in surrounding “Appalachia”, the need for Berea’s Foundation School, Academy and Normal School faded over the years, so that the only survivor is a now a substantially larger Berea College.
A unique feature of Berea was and is a student labor program that provided much of the manpower required to run the institution. Under the supervision of permanent staff, the dining halls, dormitories, offices, and yard work were all maintained and operated by student labor. In addition were several “Industries” including a bakery, broom factory, dairy, large truck garden, sheep farm, piggery, a “woodwork department” that made fine furniture, and a weaving establishment. The normal work load was two hours a day by which a student could earn as much as half or two thirds of his or her out of pocket expenses that in the 1930’s averaged only $250 to $300 per year because tuition was free! The system was very democratic because every student was required to work at least two hours a day. A fair fraction of the student body comprised so-called half-day students who worked four hours a day, earning enough to pay all expenses. I knew students who arrived at Berea with nothing but the clothes on their backs, having dropped out of school after the second grade, and worked their way through college! It is not surprising that competition to enter Berea, especially the college, was extremely keen. In order to avoid having to reject such a large fraction of applicants, the Trustees had established a policy requiring that 85 per cent of the student body come from a region of Appalachia comprising some 500 or so counties in Virginia, West Virginia, Kentucky, Tennessee, and North Carolina. The remaining 15 percent of the students came from the rest of the world. Today’s Berea is quite a different place because the growth of alternative educational opportunities in Berea’s “Territory”, has decreased demand to the point that in 1987, at my 50th reunion, I learned there were only two applicants for every opening in the College compared to 20 or 30 in my day. Part of that decrease in demand is due to a higher level of average income in that “territory” so that fewer students can show that they cannot afford to go elsewhere, a requisite for admission.
When I graduated from the Academy in 1932 I was only 15, too young, my parents thought, to go to college, so I stayed in the Academy an extra year taking courses in mechanical drawing and shorthand. I also continued the piano lessons that I had started the year before and spent enough time practicing so that I actually gave a recital during my last year in college! Alas, whatever piano skills I had have faded until now I do well to play chopsticks with my grandchildren! When I entered College with the class of ’38 I had not decided on a major but I leaned toward a science, probably because of my long affair with the Book of Knowledge, an encyclopedia for young people which my parents bought when I was around eight or nine. During the hours that I pored over them, its 20 volumes became a well worn magic carpet to new and fascinating worlds. I’ve often quipped that “I got through college on the Book of Knowledge”, a bit of rhyming hyperbole that contains an appreciable kernel of truth.
The College required at least one course in science for all students no matter what their major. Having enjoyed my chemistry course in the Academy I enrolled in Introductory Chemistry during my freshman year. It was taught by Professor Julian Capps, the senior of the two member chemistry faculty. He was a wonderful teacher who made his subject live. I was so seduced that Chemistry became my major even though Gravimetric Analysis gave me fits in my sophomore year. I repeated the lengthy phosphorous determination on three samples each of ten unknowns before I got one set of three results with acceptable agreement! It seems ironic that mass spectrometry, the center of my scientific life for the last 20 years, is really just an exercise in gravimetric analysis!
During that sophomore year an experiment in another kind of chemistry turned out to have a big effect on my chemical education. I fell in love with a girl in the junior class. Determined to catch up with her and graduate when she did, I went to summer school in 1936 at the University of Iowa because it had a 10 week session that allowed me to earn 12 semester hours of credit in Organic Chemistry under “Uncle Charlie” Raiford, and Inorganic Chemistry under Professors Jacob Cornog and Perry Bond. All in all it was a very hot and grueling summer. The romance that had blossomed in the spring and sent me off to summer school, withered the following fall. However, because I would be within two courses of finishing my degree requirements by end of that year, my third in college, I managed to get classified as a Senior and became a provisional member of Class of ’37 at the June commencement, the objective inspired by a young man’s fancy of the previous spring. My courses in chemistry thus far had required only some facility in simple algebra. Straight A’s in all Prof. Peck’s courses in the Academy (plane geometry, advanced algebra, solid geometry and trigonometry) had excused me from freshman math in college, all that was then required for a major in chemistry.
Fortunately for my future our next door neighbor, George Bent, was visited by his brother Henry, then an assistant professor of chemistry at Harvard. When Henry discovered that I was contemplating graduate work in chemistry, having had no college math, he was aghast and spent a couple of hours impressing me with the importance of mathematics in chemistry. As a result I spent much of my third and last year of college immersed in that subject. Fortune had tossed me another favor in the form of Berea’s new curriculum based on four 9 week quarters instead of two 18 week semesters. So-called “intensive” courses met 6 hours a week for nine weeks while “running” courses met 3 hours a week for eighteen. Thus, a standard full load of l5 class hours per week comprised two intensive courses and one running course, an arrangement which I heartily endorse. One major advantage is that a student takes only three courses at any one time instead of five. It is much less distracting to be studying three subjects at a time rather than five. The particular advantage for me was that the calendar time for a three credit-hour course was cut in half so that both Course B, and its prerequisie Course A, could be completed in two nine week quarters instead of two eighteen week semesters. Thus, in my senior year I was able to take Analytic Geometry, Differential Calculus, Integral Calculus, and Complex variable, all in that same year! It was pretty heavy going, but it enabled me to survive in graduate school where I took one more undergraduate course in Advanced Calculus. The record seems to say that I have also survived after graduate school but a lack of mathematical skills has been a great handicap throughout my life.
Though I had taken part in the June Commencement exercises at Berea in 1937, I still had course requirements to complete before I could get my degree. I fulfilled those requirements at Purdue University that summer by taking Physical Chemistry with lectures by Roy Newton and laboratory under Hershel Hunt, along with a course in Chemical Microscopy under Ed F. Degering.
During that hectic last year in college I had applied to several universities for graduate study in chemistry. Yale and Northwestern responded with offers of teaching assistantships that would meet most of my expenses. I first leaned toward Northwestern but, for reasons I won’t take space to enlarge on, I finally decided on Yale. That decision delayed by half a century my meeting Malcolm Dole who in 1937 was a young assistant professor of chemistry at Northwestern. It was the experiments he reported much later (in 1968) that changed the course of my scientific life.
At Yale I found myself in a new and different world. I had done time in two much larger universities and one much smaller college but I was completely unprepared for, and awed by, the splendor of Yale’s campus. Its architectural theme has been disdainfully dubbed “Fifth Avenue Gothic” by the self-styled experts who sneer at such expensive imitation of the old world. But a wide eyed small town youth from Kentucky found it all fascinating and loved to wander around and through the gardens, gargoyles and gates that were scattered throughout the campus. To me it was rewarding recreation to discover and explore the little nooks and courtyards where one could sit in the shade of a tree completely oblivious to the cacaphony of a busy city, only a few yards away but completely shut out by the thickest walls and heaviest masonry I had ever seen. Sterling Library, then said to be the largest in the world with open stacks, became a favorite haunt where I spent many winter weekend afternoons with a good book and an apple or a candy bar. My room in the Hall of Graduate Studies looked out on a forest of chimneys, slate roofs, and Gothic towers that resembled a Hollywood version of medieval London. I ate in an elegant dining hall, complete with menus and waiters – twenty one meals per week for a now incredible eight dollars! Even so, to save money I took advantage of an option in the board contract and “signed out” every week for dinner on Friday night along with breakfast and lunch on Saturday. This “fasting” saved me $1.15 every week and probably was good for my figure! My assistantship paid a total of $850 for nine months, of which $350 went for tuition and fees. By such frugalities as the weekly “fast” I could make the remaining $500 cover most of my other expenses. My parents were horrified when they later learned of my miserly existence at Yale but I was determined to get along without their support.
On the appointed day in September the six new graduate students in physical chemistry met in Sterling Chemistry Laboratory with Professor Herbert Harned, the ranking faculty member in that subject whose colleagues included Lars Onsager, Benton B. Owen, Rodney Smith, George Murphy, John Vance, and Gosta “Gus” Akerlof. With little or no ceremony, no introductions and no opportunity to ask questions or indicate preferences, we were assigned to advisors. I recall “Herbie” as he was known but not addressed, saying to me: “Fenn, you might as well go with Gus.” Thus, began what blossomed into a close friendship that lasted until the day Gus died, many years later, in Princeton.
The Yale curriculum for chemistry graduate students in those days was pretty cut and dried. During the first year we took four courses, spent 12 to 14 hours per week as assistants in undergraduate labs and the rest of the time getting our feet wet in the laboratory on what would become our dissertation research. At the end of that first year the faculty, on the basis of course grades and day to day observations, decided whether or not each student was Ph.D. material. Those for whom thumbs were down were given a Master’s degree and dismissed. The others continued, almost always receiving a Ph.D. after two more years. The second year was much like the first except that we took only a couple of courses and spent much more time on our research projects. At the beginning of the third year we had to take a two day written comprehensive exam. While I was a student, all who took that exam passed it so I don’t know what the consequences of failing would have been. One very big difference in the third year was that we could no longer be teaching assistants but were expected to spend full time on research. Thus, unless we were lucky enough to win one of the two fellowships available to third year students, we were on our own financially. My solution to this problem was to get married, not to my sophomore flame but to Margaret Wilson, who became supervisor of my student labor at Berea while I was working in the Registrar’s Office. She was a beautiful woman by whom I (then in the tenth grade!) was smitten the first day she started work as Assistant Registrar. Always ten years older than I she seemed to be so far beyond my reach that I never dreamed she could ever be more than the close friend and confidante that she became. I had escorted her to several events while I was in college and when I was home after I graduated. Romance bloomed while I was at Yale and she finally agreed to become my bride at end of my second year. She also became my “fellowship” during that third year at Yale, supporting us both on the 50 cents per hour she earned at various odd jobs. To this day I marvel that the daughter of a very conservative minister had the courage to ignore the disapproval of her parents and the inevitable elevation of eyebrows by everybody, everywhere, at our flagrant departure from the social norm. One of my closest friends told us decades later that he had been sure our marriage would not last more than a year or so. In fact it was not until 1992 that I lost my bride of 53 years in a New Zealand car crash. She was then 85 but looked and acted ten years younger than the husband she had “snatched from the cradle.”
The plain truth is that in matters of matrimony this society’s norms are genetically out of joint. Women on average live about ten years longer than men and yet become the wives of husbands who are several years older. That is why widows greatly outnumber widowers. Some say that this imbalance in life expectancy will be redressed as women increasingly become exposed to the stresses of employment in the workplace. I am much more inclined to believe the geneticist who told me that the female of the species lived longer than the male because she is fundamentally tougher and more resistant to the ravages of age. In choosing a mate men should follow the advice of Ben Franklin in his letter of advice to a young man on choosing a mistress. His message? “be sure she is older than you are!”
Only in choosing a graduate school did I exert any influence on the research I would do for my thesis. That influence was unwitting because I knew nothing of the faculty research interests at either Yale or Northwestern. Indeed I had only the vaguest idea of what graduate study and research were all about. Thus, I felt neither joy nor apprehension when I was summarily assigned to Gus Akerlof’s group. Most of the research by Harned and his colleagues was on the properties of electrolyte solutions, the more dilute the better. So narrow was this focus that when I left Yale I halfway believed the old cliche that “Physical Chemistry was the study of slightly contaminated water.” Gus was a bit of a maverick because he had an interest in concentrated solutions. In essence what I had to do was measure the potential difference between electrodes of silver-silver chloride and platinum-hydrogen in solutions of HCl with molalities from 0.01 to 10.0 in solvents comprising methanol in water at concentration intervals of 10 per cent from zero to ninety, at temperatures every ten degrees from zero to 50 Celsius. As I remember the total number of acceptable emf measurements was around 3000, taking into account that each measurement was made in duplicate. Thus, my routine every afternoon for most of two years was to prepare three pairs of cells with both cells of each pair containing the same solution. The cells were suspended in a large water bath or “thermostat” and allowed to equilibrate over night (i.e. with hydrogen bubbling over the Pt electrodes.) If by the next morning the emfs in each pair of cells agreed to within some forgotten small fraction of a millivolt, then I would haul some 80 pounds or so of ice from the basement to lower the bath temperature to near 273 K. The rest of the day would be spent recording emf values for all six cells at every ten K as the thermostat was heated to 313 or 323 K depending on the vapor pressure (i.e. the methanol content) of the cell solvent. All the results were fitted by least squares to a quadratic curve for the dependence of emf on concentration. The least square calculations were all carried out on a hand-cranked Monroe Calculator, except when I was lucky enough to grab the one electrically driven machine in the calculating room. Clearly, I was less than inspired by this introduction to “original research”. The experiments were a boring chore with few redeeming features. The results contained no surprises and nothing of much interest to anyone, least of all me. It was some years later before any of them found their way into the literature and then only as part of a table in a review article that Gus had been asked to write. My dissertation attested to the sterility of that project, consisting as it did of 45 pages of tables with only three pages of text! Although this first experience in “original research” shattered some illusions, my three years at Yale were a rewarding experience. I made many long lasting friendships with both fellow students and faculty. The Physical Chemists were a very congenial group and Herbie Harned was really a very interesting man, except in the class room. He came by almost every lab almost every day to chat with us students about almost everything. Sometimes his visits were inconveniently long and would interfere with experiments. One of the older graduate students had told me that Herbie would run for cover if he were asked to help, for example by holding a light or a tool during some adjustment. That ploy worked like a charm and saved me many hours. Actually, the Harneds were very kind and warm hearted people. They held an open house every Friday night which both students and faculty felt obliged to attend more or less regularly. More often than not we ended up around the piano in a group sing-along with Roger Bates, then a Sterling Fellow and accomplished musician, at the keyboard.
I also learned a lot about life and lore outside of chemistry, e.g. to play bridge, to drink beer and to smoke a pipe during the mostly dull seminars every Tuesday night. I had many interactions with many interesting people. The weekly university calendar was filled with provocative events ranging from seminars to sermons and concerts to contests. My perspectives were stretched by memorable if abstruse encounters with great minds, including lectures on resonance by Linus Pauling, and two required courses in statistical mechanics under Lars Onsager (known to the students as Norwegian I and II!). Gus and his wife Rosalie, who was the sister of Joe Hirschfelder, looked after his students with great care and affection, making their house our home away from home. Rosalie took my new bride under her wing, showering us newlyweds with almost too much attention. I was extremely fond of Gus and most grateful for his always calm demeanor and seeming imperturbability. He strongly disapproved of marriage for graduate students but when I told him I wanted to take a wife he protested not at all and simply said “OK, we take it from here.” He and “Magee” as my wife was later known, became extremely fond of each other and he later told me many times that marrying her was the smartest thing I ever did, an opinion shared by everyone who has known us both. All in all, my time at Yale was happy and rewarding but I left with a much diminished interest in “scholarly research”, and no desire to return. I often think about how surprised and pleased Gus would have been to know that six decades later his son George Akerlof would share the 2001 Nobel Prize in economics and his student John Fenn would share the 2002 Prize in Chemistry!
My immediate and exciting prospect after graduation from Yale was a job in the research department of the Phosphate Division of Monsanto Chemical Company in Anniston, Alabama. The starting salary was $2700 per year, then the going rate for new PhD’s in industry and a mind-boggling jump from the $1000 or so a year Magee and I had been living on. I couldn’t understand why my mother laughed when I once remarked that I didn’t know how we could spend so much money!
Anniston, the county seat of Calhoun county and “Cast Iron Pipe Capital of the World”, was located halfway between Atlanta and Birmingham. Living there was my introduction to the deep south where cotton still grew and the Confederate Flag still flew. It was the home of Fort McClellan, a long time army base that was already gearing up for America’s forthcoming participation in World War II. Two of my mother’s brothers had been medical officers at McClellan during World War I and two of our closest friends from New Haven passed through there after being drafted in the wake of Pearl Harbor. We had the chance to compensate them a wee bit with an occasional home-cooked meal. I was more fortunate because the army was not yet drafting husbands.
Monsanto had recently acquired the Anniston Plant when it bought Swann Chemical Company started by a legendary character named Theodore Swann. He had pioneered the production of phosphoric acid from elemental phosphorous shipped in by tank car from a plant in Columbia, Tennessee where it was produced by smelting “phos rock” in an electric furnace. At Anniston the phosphorous was burned in air to produce its pentoxide which could be hydrated to form acid of any desired concentration. Major customers for the acid per se included the soft drink industry, especially the Coca Cola Company in nearby Atlanta. According to local folk lore, the first tank car of food grade acid to be delivered was filtered through laboratory Buechner funnels because the filtration plant was not ready in time to meet the deadline on the sales contract! Much of the phosphoric acid left the plant in the form of sodium and potassium phosphates that among other applications were widely used as soap builders, water conditioners and in food products such as baking powders.
The other main product line, which some said was the main reason Monsanto bought Swann, comprised biphenyl and its chlorinated derivatives known as Arochlors, the now notorious PCBs. Biphenyl was produced by bubbling benzene vapor through large baths of molten lead at a temperature of around 800°C, as I remember. Liquid metal as a heating agent provided a rapid rise and close control of the temperature, thus minimizing formation of byproducts which mostly comprised ortho, meta and para diphenyl benzenes. The various Arochlors were characterized by their chlorine content which ranged from 10 per cent to 60 per cent by weight. One of their main uses was as transformer oils because they had very good heat transfer characteristics, would not burn and were very inert. Indeed, they were so inert and sticky that the chemists insisted that if Arochlors ever got into a laboratory some of it would remain forever! Because it was so inert we practically bathed in the stuff, never dreaming that it might be toxic. General Electric dumped PCB wastes into the Hudson River for many years and is now resisting Federal orders to dredge it all up. Recently, the press has reported growing anger in Anniston and threats of legal action as new surveys have shown the extent to which PCBs from the plant where I worked have contaminated the soil and waters around the town. My own substantial exposure seems to have had no ill effects, but I daresay that even today, sixty years later, the PCB content of my fatty tissue would horrify the EPA! There is no doubt that PCBs in the environment accumulate in the fatty tissues of mammals and fish. The evidence of resulting toxicity is less convincing. I remember a paper by an Australian chemist claiming that the only documented evidence of significant toxicity stemmed from two cases in which PCB’s, used as the heat transfer medium in the heating coils of deep fat fryers, leaked into the fat. The resulting reaction produced highly toxic benzo-compounds whose identity I have forgotten. I do know that sixty years ago I practically bathed in the stuff while operating a pilot plant in which PCB was produced in the vapor phase by reaction of biphenyl with HCl and air over a copper catalyst in a variation of the old Deacon process for producing chlorine by the oxidation of HCl. Occasionally I would get a slight skin rash, as did the other operators, but I have detected no lasting effects.
After I had been at Anniston for a year, James W. Mullen II., a young Ph.D. organic chemist from Princeton came aboard. He and I became good friends, sharing a growing dissatisfaction with the way things were being run at work. Jim insisted that someday he was going to start a research company and that when he did he wanted me to join him. Talking about what we might do was fun but it all seemed like pipe dreaming to me. Having become increasingly disenchanted with the work situation we both resigned and left Anniston on the same day in 1943. Jim went to Bell Labs in New Jersey. I took my family, which now included an 18 month old daughter, Marianne, to Wyandotte, Michigan where I had obtained a job in the research department of Sharples Chemicals, a relatively small producer of alcohols, amines, esters and other derivatives of amyl chloride produced by the chlorination of pentane. One day in June of 1945 a letter came from Jim saying that he had finally started his company and wanted me to join him. I took a train down to Richmond to find out more. At Bell Labs he had become involved in Project Bumblebee, a large scale effort by the Navy to develop a ramjet-powered anti-aircraft missile for the fleet. The ramjet depends upon ram pressure from high speed flight to compress air enough so that its expansion after heating by combustion could provide useful thrust. It was clear that supersonic flight velocities would be required to achieve substantial thrust but because there was little or no information on drag at such velocities, nobody knew whether that thrust could equal or exceed the drag. The Project name, Bumblebee, derived from a bit of aeronautical badinage to the effect that by all the laws of modern aerodynamics it is impossible for a bumblebee to fly, but the bumblebee doesn’t know any aerodynamics so it goes ahead and flies anyway!
From flames to flying elephants
Experiment, Inc., the name Jim Mullen gave his company, prospered over the next seven years. To watch and participate in its growth, from a total of three employees when I arrived to perhaps 45 or so when I left in 1952, was an exciting experience. Most of its business was based on R&D contracts with government agencies for which the central theme was combustion and propulsion but we did pursue some commercial ventures. There were some important personal dividends from my investment of time at Experiment. One was that I learned a little bit about the dynamics and thermodynamics of compressible flow, a newly popular area of research spawned by the advent of jet propulsion and sometimes referred to as “Aerothermodynamics”, or “Aerothermochemistry” if changes in chemical composition were involved. Another personal dividend was the opportunity to do some research in combustion, both applied and fundamental. Jim was a strong believer in publishing our results. Thus the first research publication of my life was a paper on ignition in high speed flow, co-authored by Jim and a junior colleague, M.R. Irby. That paper appeared in 1949, almost a decade after I left graduate school1. Consequent to several more papers, visits to other labs, and attendance at various meetings, I began to get some recognition in the Combustion Community. Moreover, in an about-face from my feelings at Yale, I found I enjoyed doing research and communicating with others about my findings and theirs.
My life in Berea had made me think that I would someday like to become a college professor. But I had also thought that I should get some of the realworld industrial experience that had seemed to help make Julian Capps, my chemistry professor at Berea, such an effective teacher. That desire to join a college faculty had faded somewhat at Yale but was revived a bit during my experience at Experiment, Inc. We obtained a number of provocative results on some properties of flames, in particular on stabilizing them in high velocity flows. Both Jim and the sponsors of our studies were happy to have us attend technical meetings and to publish results of our studies, (so long as they didn’t involve classified information.) Consequently, I got to know, and become known by, a number of people in the combustion community. One result of this new and limited “notoriety” was an offer from Princeton University to serve as Director of Project SQUID, a program of pure and applied research in “those fields of science relating to jet propulsion” including combustion, fluid flow and heat transfer. Financed by the Office of Naval Research (ONR) and administered by Princeton, Project SQUID was named after the jet-propelled decapod of the sea. (That name had additional but unpremeditated relevance in that both the animal and its namesake secreted ink, the former in clouds that confused predators, the latter in print in reports that confused readers!) SQUID’s organizational structure comprised a prime contract between ONR and Princeton, with subcontracts at any one time between Princeton and from 12 to 20 or so university and industrial laboratories. The overall purpose was to cultivate interest and activity by students, faculty and other investigators in combustion, fluid flow and heat transfer.
One reason for my interest in Princeton was that by then I had learned that research could be a lot more fun than it had been when I was a student at Yale. Another reason was that my Yale mentor, Gus Akerlof, had set up a laboratory in its newly established James Forrestal Center to develop, with Navy (but not SQUID) support, an electrical discharge process for the production of hydrazine, an attractive rocket propellant. Gus had once visited me in Richmond and in fact had put my name in the pot at Princeton when he learned that the previous director of SQUID was leaving. One of the “other reasons” for my interest was that Jim Mullen and many of his friends, were Princeton graduates. After seven years among those fiercely loyal alumni I had been brainwashed to the point of believing that maybe Old Nassau really was the “best old place of all.” Moreover, as I have already noted, my life in Berea had long made me think that I would enjoy becoming a teacher and living in a college community.
At Princeton I would report directly to Sir Hugh Taylor, long-time Chairman of Princeton’s Chemistry Department, then Dean of the Graduate School and Chairman of the University Research Board. The position was “with rank of Professor” and the Dean assured me that I would be welcomed as an active member of the academic community. And so, after much soul searching, Magee and I decided to leave the “Cradle of the Confederacy” for what was and is often called “the northernmost southern town in the country”. That epithet stemmed from the substantial numbers of Princeton students that over the years have come from below the Mason Dixon line. Before the Civil War it had become customary for students from the south to celebrate graduation by freeing their personal servants. An interesting result is that Princeton has a much larger black population than do other towns of comparable size in that part of the country.
My new job turned out to be very interesting and highly educational. I had to travel a lot but was able to visit many laboratories and meet well known scientists in many fields all over the country. I got to know the people in ONR pretty well and my circle of acquaintances included people in ARO (Army Research Office) and AFOSR (Air Force Office of Scientific Research) both of which were providing some support for Project SQUID and were represented on its Steering Committee. ONR had a branch in London (ONRL) that maintained a rotating corps of 12 to 15 Scientific Liaison Officers whose job was to promote interaction between scientists and engineers in Europe and America by visiting laboratories and attending scientific meetings. They would get to know the investigators, learn about what they were doing, help them establish contacts with their counterparts in America, arrange visits, find items of equipment, assist with travel arrangements, and the like. Research laboratories in Europe were beginning to recover from the trauma of World War II and they welcomed the communication links and the help with their needs that ONRL could provide. The SQUID office had occasionally been involved in making travel and visiting arrangements for some of the European scientists who came to America under ONRL’s auspices. After I had been at Princeton for about three years I was invited to serve a year as an ONRL Liaison Officer in the areas of combustion and propulsion. My bosses in ONR Washington approved and I persuaded John Scott, who had just finished his Ph.D. in Aeronautical Engineering at Princeton, to mind the store at SQUID while I was gone. Thus, Magee and I and the children, then 9, 11 and 13, went to London for the calendar year of 1955. And what a wonderful year it was! The children still insist that living London was the high point in the Fenn family fortunes which have been going down hill ever since! I share some of those sentiments because that year was also a marvelous experience for the parents. The kids were in school so Magee was free to roam London during the day. I visited many laboratories in many countries, made many friends, and learned “more things than had been dreamt of in my philosophy.”
Among these “things” was my stumbling on to something that was to set the stage for the rest of my scientific life. Up to that time flames and their behaviour were the focal point of almost all efforts to elucidate the kinetics and mechanisms of the important reactions in high temperature combustion. The general approach was mostly based on attempts to measure and interpret flame characteristics such as propagation velocity, flammability limits, ignition temperatures and the like. I had become convinced that flames, with their extremely high gradients of temperature and composition, were much too complex to be useful as stages for the study of those reactions. I often recalled a comment by Philip Rudnick, professor of Physics at Vanderbilt, who was active in the propulsion Panel of Project Bumblebee. He said that the more he learned about flames the more he was convinced that they were organisms whose study properly belonged in the province of biology! I’m sure that view strikes a responsive note in many investigators who have been exasperated by the vagaries of flame behaviour that seem to give them a life of their own. Indeed, the analogy can be extended. Flames, like organisms, are born, need fuel and oxidant for nourishment, grow and multiply to the extent that those necessities are accessible, and are poisoned unto death when immersed in their own waste products! Not only is their structure and behavior complex but the conversion of reactants to products involves sequences of so many reactions over such short distances that to determine how the composition and temperature changed with distance (i.e. time) in real flames, i.e. the relation between their “fine structure” and their behaviour, was not experimentally feasible with the tools and techniques then available.
Thus, for some time I had been somewhat naively musing about whether and how it might be possible to study chemical reactions by the same approach that physicists so successfully use in the study of nuclear reactions. They build accelerators to produce beams of atomic nuclei with energies of megavolts to gigavolts with which they bombard stationary targets comprising collision partners of interest. They then characterize the products from reactive collisions by analyzing the post-collision trajectories of the product particles as measured by appropriate detectors, e.g. bubble or cloud chambers. I had been introduced to the possible uses of molecular beam scattering experiments in chemistry by Professor I. (Izzy) Amdur, a colleague of the Professor Frederick Keyes at MIT whose elegant measurements on the transport properties of gases were being supported by Project SQUID. Amdur was using beam scattering experiments to characterize the intermolecular potentials of molecules. His approach was based on electrostatic acceleration of ions to relatively high energies and then neutralizing them by charge-exchange collisions with neutral molecules for which the cross-sections are much larger than those for momentum exchange. In this way he could produce beams of neutral molecules with translational energies equal to those of the incident ions. Space-charge effects give rise to the Childs-Langmuir law by which the maximum intensity (current density) of an ion beam is proportional to the square root of its energy. Consequently, neutral beams with intensities high enough to provide good signal/noise in a scattering experiment, must perforce have energies substantially higher than the intermolecular potentials that Amdur was trying to probe. His solution to this problem was to locate his detector so that it would “see” only the post-collision beam molecules whose trajectories differed by only a very small amount from their pre-collision values. This “small-angle scattering” approach allowed him to exploit the high intensities obtainable with beams of high energy molecules (in laboratory coordinates) in the study of molecule-molecule interactions at low energies in center-of-mass coordinates of the colliding partners. Unfortunately, that approach did not seem to lend itself to the study of reactive collisions.
When I went to ONR London in late 1954, nobody had successfully carried out reactive scattering experiments with molecular beams on any chemical reaction, even one with a negligibly small activation energy. Moreover, activation energies for many combustion reactions were known to be as high as 30 kcal per moltwo or more. The energies of beam molecules from conventional effusive sources are always limited by the temperature at which the source can be operated. The average translational energy of an oxygen molecule from a source at 3000 K would be only about 10 kcal/mol or 1.2 eV, (but that temperature is well above the melting point of most materials from which the source might be made!). Any polyatomic molecules of interest in combustion would completely decompose even at much lower temperatures. In sum, the collision energies that can be reached simply by raising beam source temperatures are severely limited. Even so, the possibility of bringing about reactive collisions by colliding a beam of reactant molecules with other gaseous molecules had been contemplated since the late 1920’s. In spite of numerous attempts, no really convincing results had been obtained when we left for London at the end of 1954. However, shortly after we returned to Princeton in early 1956, the age of reactive scattering experiments with molecular beams dawned when Datz and Taylor reported that intersecting beams of K atoms and HBr molecules produced detectable amounts of KBr.2 These results gave some substance to my musings but there was a fundamental problem with the idea of applying the same method to combustion reactions. Activation energies for K+HBr are negligibly small. For many of the most interesting reactions in combustion the activation energies were thought to range from 0.5 to 2.0 or more eV, much much higher than for the reaction studied by Datz and Taylor. Amdur’s charge exchange technique could easily produce beams with much higher energies but because of space charge effects could not provide useful intensities at energies as low as a few eV. Nor did Amdur’s ploy of looking only at small angle scattering seem applicable in the study reactive scattering. To produce beam molecules from classical effusive sources with energies of even 0.5 eV would require source temperatures of 3000 K. Neither materials of construction for the source, nor reactant molecules other than atoms, could endure such temperatures. The situation thus seemed hopeless.
One day in London I was calculating rocket exhaust velocities achievable with various propellants and suddenly realized that many reactant molecules at the velocities attainable with some such propellants would have translational energies as high as two or more eV. I began to fantasize about using micro- rockets in vacuum as molecular beam sources, anticipating by 30 years an experiment we actually carried out for another purpose! I then remembered that rocket visionaries had often touted hydrogen as an ideal propellant fluid for nuclear powered rockets because its of its low molecular weight. The limiting maximum convective velocity that can be reached by a gas expanding into vacuum is equal to (Cp/MT0)1/2 where Cp is its constant pressure heat capacity, M its molecular weight and T0 its source temperature. Thus the maximum velocity reachable by helium is about 3.33 times that for argon. If the helium is “seeded” with one or two per cent argon, and if those argon atoms are swept along like dust particles in a wind storm, the translational kinetic energy of the argon atoms after free jet expansion of the mixture would be almost 10 times that of the helium atoms, if the two reached the same terminal flow velocity.
A few days later I was browsing in ONRL’s library and ran across the now famous paper by E.W. Becker and K. Bier reporting the production of intense beams of hydrogen molecules by expansion of the gas from high pressure through a small converging-diverging nozzle into vacuum3. That paper referenced the also now famous paper by Kantrowitz and Grey in the Review of Scientific Instruments which proposed the use of a converging-diverging nozzle to produce a jet of high velocity molecules in a region of low pressure.4 A small core portion of that jet would be admitted through a conical “skimmer” into a high vacuum region to form a jet of molecules. The “static” temperature of the molecules (as measured by a thermometer traveling with the gas and at the same velocity) would be very low so that the divergence of the molecular trajectories downstream of the skimmer would be very small and the velocity distribution in the direction of flow would also be very narrow. In sum, the jet would comprise a very intense beam of molecules, all traveling at nearly the same velocity! Another paper in the same issue of that journal reported a not very successful attempt by Kistiakowsky and Schlichter to reduce the Kantrowitz-Grey idea to practice.5 Both of those papers also happened to be in the ONRL library and when I read them I began to take my musings seriously. (It is said that Kistiakowsky and Schlicter were so frustrated by troubles in their experiment that when Schlicter had suffered enough to deserve a degree, they vented their frustration by demolishing the apparatus with an axe!)
I later learned that twentyfive years earlier, T.H. Johnson, a Sterling Fellow at Yale, had produced intense beams of mercury atoms by expanding the vapor from a “boiler” at several hundred torr into vacuum6. Unfortunately this truly remarkable result had been ignored because it was at odds with the dogma from Stern’s lab in Hamburg that raising the source gas pressure (density) would produce a sort of stagnant cloud of molecules at the exit of the source orifice. That mythical cloud would scatter a large fraction of the emerging molecules that might otherwise have contributed to beam intensity. Later in his career at the Franklin Institute Johnson he also found diffraction effects in the scattering of hydrogen from an LiF crystal, confirming the wave nature of particles much heavier than the electrons with which Davisson and Germer had earlier found diffraction effects. Unfortunately, some eight weeks earlier Estermann, Frisch and Stern had found the same result in Göttingen7 so Johnson’s work again went unnoticed while Stern got the Nobel Prize! An able investigator who was 25 years too early with one landmark experiment and 8 weeks too late with another, Johnson had every right to feel frustrated by the fickleness of fate.
The results predicted by Kantrowitz and Grey in 1951, having been already achieved by Johnson in 1929, were confirmed in the above-mentioned paper by Becker and Bier in 1954. They showed that convective flow of gas through a small orifice from high pressure into vacuum could indeed produce molecular beams with much higher intensities than could the effusive sources introduced and exploited to great advantage by Otto Stern and his colleagues. Moreover, these “convective” beams had much narrower velocity distributions than did their effusive cousins. Consequently, the achievable intensity within a narrow velocity interval, a highly desirable feature, could be very much higher with convective beams. What had not yet been shown, nor apparently considered, was that heavy molecules could be accelerated to suprathermal translational energies by expansion of a light carrier gas such as hydrogen or helium in which those heavy molecules were present a low levels.
When I returned to Princeton after my year in London, I found that John Scott, who had run the SQUID Office during my absence, was going to the University of Virginia as an assistant professor of Aeronautical Engineering. He was wondering what research he ought to work on and I told him about my musings on molecular beams from high pressure sources. I also told him that if he would submit proposal on such work to Project SQUID I would try my best to get support for it. When he arrived at Virginia he found a group that was already using beam scattering experiments to characterize the exchange of energy and momentum between molecules and surfaces. Their goal was to understand and predict surface heat transfer and drag for aerodynamic bodies at very high altitudes where flow was molecular rather than continuum. The high intensities achievable with beams from supersonic free jets (or “nozzle beams”, as they came to be known) would be most useful in such surface scattering experiments. Moreover, the high translational energies that seemed achievable with the seeded beams I was dreaming about, would make possible the study of drag and heat transfer in molecular flow under conditions much closer to those expected in ultra high altitude flight than those obtainable with the effusive beams they had been using. As a result of their enthusiasm and mine, the first nozzle beam system in America was built in Charlottesville, VA.8 I should really say, the first in America since the one T.H. Johnson built at Yale in 1929 that produced high intensity beams of mercury atoms but had been ignored. It now seems clear that the reason for Johnson’s success was that the walls of his system were all water cooled enough to condense most of the incident mercury atoms. The net result was an effective pumping speed orders of magnitude higher than the nominal speed of his vacuum pumps or those of any of the other molecular beam systems then in existence. (Fraser in his 1932 book on “Molecular Rays” refers to a system then being built with the “exorbitant” pumping speed of 100 liters per second9. The nominal speed of each of two 32 inch pumps that we bought “off the shelf” 45 years later was 30,000 liters per second!) The real reason for the generally observed decrease in beam intensity with increasing source gas density was scattering due not to the molecules in a “cloud” at the source orifice exit but to those in the relatively high density of background gas throughout the system due to inadequate pumping speed.
Meanwhile, I was getting more and more intrigued with the problems and possibilities of beams from free jet sources and eager to become more directly involved. I had by this time learned that in spite of Dean Taylor’s rosy assurances I could not become an active participant in academic research unless I had some direct association with a department. SQUID had been supporting research on two-phase flow by Shao Lee (Charlie) Soo, a young assistant professor of Mechanical Engineering. During a visit to Soo’s lab one day I met Robert Drake, the new chairman hired to refurbish that department’s image by expanding its research activities. I described my wild ideas on free jet beams and said: “If I write a proposal and get some support will your department provide a home for the project?” Bob had done his doctoral research in rarefied gas dynamics at UC Berkeley. Familiar with the molecular perspective on gas dynamics he immediately said yes. I prepared a proposal on which I was listed as a “Consultant” because I was not on the faculty and under university rules could not be a Principal Investigator. That role was assumed jointly by Bob Drake and Michele Boudart, my next door neighbor and close friend who was interested in chemical kinetics and catalysis and was on the faculty of Chemical Engineering. I had been discussing my beam ideas with him and he too found them intriguing. The proposal was submitted to NSF who sent it for review to Immanuel Estermann, a co-author with Stern and Frisch of the landmark paper on diffractive scattering of hydrogen from LiF, one of the two papers cited when Stern won the Nobel prize in 1943. Estermann had become Director of ONR’s Material Sciences Division (whose Power Branch was responsible for Project SQUID) and was thus my ultimate boss. He took his reviewing seriously and spent a day in Princeton discussing the proposal with me. (The fact that his daughter was then living in Princeton was no doubt something of an inducement for him to make the trip from Washington.) He had visited Becker’s laboratory in Marburg, Germany and was aware of the difficulties Becker had encountered in fabricating the tiny converging-diverging nozzles prescribed by Kantrowitz and Grey. As he took his leave he said: “John, I’m afraid this research may be too difficult to pursue with graduate students but I think it is important so I’m going to recommend support.” He apparently was as good as his word because NSF gave us the money we had sought and we began work in the fall of 1960. As it turned out, Estermann’s fears about the difficulties of making very small Laval (converging- diverging) nozzles were groundless. The Marburg group discovered empirically, that beam intensities became higher when the diverging section of the nozzle was cut off!10 The lower intensities obtained with a diverging nozzle are due to viscosity effects which Kantrowitz and Grey had ignored in their analysis. Dave Miller’s group in San Diego, later showed experimentally that for most practical purposes the jets from a simple orificea (hole in a flat plate), a simple converging nozzle, or a long tube, had almost identical properties as long as the flow was “choked”, i.e. reached sonic velocity, at the exit plane of the nozzle, tube or orifice.11
This autobiography/biography was written at the time of the award and later published in the book series Les Prix Nobel/ Nobel Lectures/The Nobel Prizes. The information is sometimes updated with an addendum submitted by the Laureate.
John B. Fenn died on 10 December, 2010.
Nobel Prizes and laureates
Six prizes were awarded for achievements that have conferred the greatest benefit to humankind. The 12 laureates' work and discoveries range from proteins' structures and machine learning to fighting for a world free of nuclear weapons.
See them all presented here.