Popular information
Information for the public:
A powerful tool for chemists (pdf)
Populärvetenskaplig information:
Ett slagkraftigt verktyg för kemister (pdf)
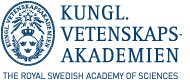
The Nobel Prize in Chemistry 2010
There is an increasing need for complex chemicals. Humanity wants new medicines that can cure cancer or halt the devastating effects of deadly viruses in the human body. The electronics industry is searching for substances that can emit light, and the agricultural industry wants substances that can protect crops. The Nobel Prize in Chemistry 2010 rewards a tool that has improved the ability of chemists to satisfy all of these wishes very efficiently: palladium-catalyzed cross coupling.
A powerful tool for chemists
At the end of the 1980s, scuba divers in the Caribbean Sea collected the marine sponge Discodermia dissoluta. At a depth of 33 meters (108 feet) they found a little creature that lacks eyes, a mouth, stomach and bones. At first sight it appears primitive, but its inability to escape enemies has turned Discodermia dissoluta and other marine sponges into masters of chemistry. They have a remarkable ability to produce large and complex chemical molecules that are poisonous and that prevent other organisms from exploiting them.
Researchers have discovered that many of these poisons have therapeutic properties; they can function as antibiotics or as anti-viral or anti-inflammatory medicines. In the case of Discodermia dissoluta, the first laboratory tests revealed that the substance discodermolide could in the future be used as a chemotherapy drug. Among other things, it stopped cancer cells from growing in test tubes.
Removes a significant obstacle to progress
After more in-depth studies, scientists have been able to demonstrate how discodermolide defeats cancer cells in the same manner as Taxol, one of the most commonly used cancer drugs in the world. Finding a substance with such huge potential is a thrilling experience in itself, but without the discoveries being rewarded by the Nobel Prize in Chemistry 2010, the story of discodermolide would probably have ended there (figure 1). Progress would have come to a halt due to a lack of material, as it is not possible to develop medicines based on a substance found only in small quantities deep in the Caribbean Sea. However, with the addition of palladium-catalyzed cross-coupling reactions to the chemist’s toolbox by Richard F. Heck, Ei-ichi Negishi and Akira Suzuki, scientists can now artificially produce discodermolide. Negishi’s variant of the reaction was used as a central step in its synthesis. Other scientists have subsequently optimized the process and managed to obtain sufficient quantities of discodermolide to begin clinical testing on humans suffering from cancer.
Only the future will tell if discodermolide turns out to be a life-saving drug. In any case, it is one of many examples of how naturally occurring chemicals inspire the work of chemists. Common to all molecules in living organisms, so called organic molecules, is the fact that they consist of a more or less complex skeleton of carbon atoms. Carbon-carbon bonds are the basis of the chemistry of life itself, and its importance to chemists is well illustrated by the fact that the subject matter has now been rewarded with a total of five Nobel Prizes. The previous four are: the Grignard reaction (1912), the Diels-Alder reaction (1950), the Wittig reaction (1979), and olefin metathesis (2005).
Palladium – point of rendezvous for carbon atoms
The palladium-catalyzed cross-coupling reaction is unique since it is possible to carry it out under mild conditions and with very high precision. Previously, chemists had to kick-start the chemical reaction between two carbon atoms using reactive substances. Such substances do their job, but the carbon often also reacts with other atoms leading to the creation of unwanted by-products. When chemists want to create large molecules such as discodermolide they build up the molecule in several steps. If the wasteful generation of by-products in each reaction is too large, there will eventually not be any material left to work with.
In the palladium-catalyzed reaction, scientists use the element palladium as a point of rendezvous for the carbon atoms. They attach to the palladium atom and are thus positioned close enough to each other for the reaction to start. Palladium functions as a catalyst. It takes part in and facilitates the process, but is not itself consumed.
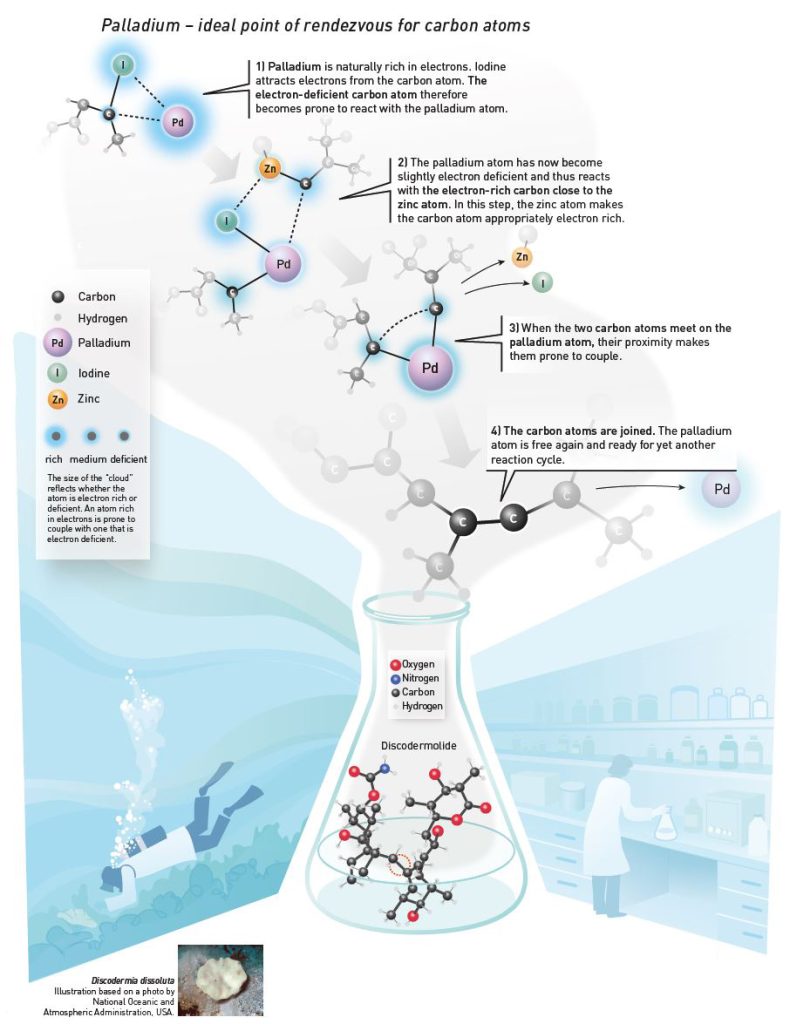
Inspired by industrial progress
The possibility of using palladium as a catalyst began to arouse interest during the 1950s. At that time, a German chemical company, Wacker Chemie AG, began to use palladium in order to transform ethylene to acetaldehyde, an important raw material used in paint binding agents, plastic softeners and in the production of acetic acid.
Richard Heck was working for an American chemical company in Delaware, and as the chemical industry got increasingly curious about the successful Wacker-process he began experimenting with using palladium as a catalyst. In 1968 he published his successful work in a series of scientific articles. Among other things, he was able to link a ring of carbon atoms to a shorter fragment of carbon in order to obtain styrene (figure 2), a major component in the plastic polystyrene. Four years later he had further developed his reaction and today the so-called Heck reaction is one of the most important for creating single bonds between carbon atoms. It is, for instance, used in large-scale production of the anti-inflammatory drug naproxen, the asthma drug montelukast and to produce a substance used in the electronics industry.
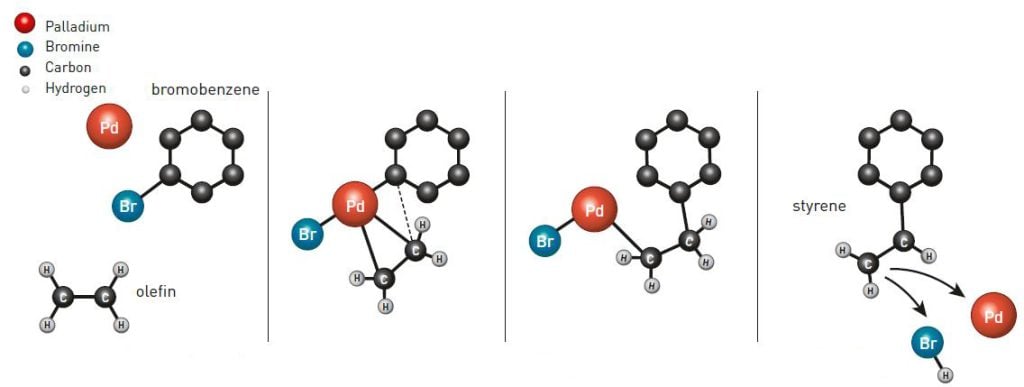
Eight – a magic number in organic chemistry
In order to understand the importance of Richard Heck’s discovery we need to plunge into the world of atoms; into the cloud of electrons that circle around the atomic nucleus. Electrons are often illustrated as small particles spinning around the nucleus of an atom. But the electron is actually more akin to a negatively charged cloud that envelopes the positively charged nucleus.
Around the nucleus there are several different layers of electron clouds and the larger the atom the greater the number of layers. Chemists are interested in the number of electrons found in the outermost layer, because all chemical reactions are essentially about the need of the atom to make this layer complete. In the smaller atoms central to organic chemistry, e.g. carbon, oxygen and nitrogen, there should always be eight electrons in the outermost layer. Eight is the magic number in organic chemistry.
In its naturally occurring form, the carbon atom has only four electrons in its outermost layer. Therefore it strives to attach to other atoms so that electrons can be shared via chemical bonds. For example, in the simplest organic molecule, methane, the carbon atom shares electrons with four different hydrogen atoms. In this way its outermost layer becomes complete and the atom is satisfied.
Fooling a satisfied atom
When chemists build complex molecules such as discodermolide they take a short-cut and use pre-existing smaller molecules as building blocks. However, linking these small molecules is easier said than done. The carbon atoms in the smaller molecules are already sharing electrons with other atoms in the molecule; they already have eight electrons in their outermost electron cloud and are thus stable. They have no reason to react with a carbon atom in another molecule.
The task of the chemist is to try to wake the carbon atom up and make it more inclined to react with another carbon atom. Victor Grignard, Nobel Laureate in Chemistry in 1912, found a solution to this problem. Using various chemical tricks he coupled a magnesium atom to a carbon atom that he wanted to make more reactive. Magnesium has two electrons in its outermost layer, and would prefer to get rid of them both. In the substance called the Grignard reagent, the magnesium atom therefore pushes the two electrons in the bond so that they mostly end up on the carbon atom. Electrons are thereby added to the outermost electron cloud of the carbon atom, but at the same time an imbalance is created between the positively charged atomic nucleus and its negatively charged electron clouds. The carbon becomes unstable, and therefore seeks another atom with which to bond.
Precision – the key to gigantic molecular constructions
The Grignard method of coupling carbon atoms has been enormously important in chemistry. But when it comes to creating large and complex molecules, the method has its limitations. The carbon atom in the unstable Grignard reagent does not behave predictably. When the reagent has several different carbon atoms to react with, too many unwanted by-products are created.
The palladium-catalyzed cross-coupling reaction solves this problem and provides precision in the process. When the carbon atoms meet on a palladium atom, chemists do not need to activate the carbon atom to the same extent. This entails fewer by-products and a more efficient reaction.
Instead of the Grignard reagent Richard Heck began to use chemical compounds called olefins. In an olefin the carbon atom is naturally slightly activated and when it binds to the palladium atom it becomes even more likely to react with another carbon atom.
In 1977, Ei-ichi Negishi developed a variant of the Grignard reagent when he substituted magnesium for zinc. The carbon becomes less reactive when using zinc, but the zinc atom transfers the carbon atom to the palladium atom. When the carbon atom subsequently meets another carbon atom on the palladium atom, they are then prone to couple (figure 1).
Two years later, Akira Suzuki used the element boron. It is the mildest activator so far and is even less toxic than zinc, which is an advantage when it comes to large-scale applications. For instance, Suzuki’s reaction is used in the commercial synthesis (thousands of tons) of a substance that protects agricultural crops from fungi.
Today the Heck reaction, Negishi reaction and Suzuki reaction are of considerable importance to chemists. One of the most spectacular examples where palladium-catalyzed cross coupling has been used is in the test tube creation of palytoxin – a dinosaur in the chemical world (figure 3). It is a naturally occurring poison that was first isolated from a coral in Hawaii in 1971. Palytoxin consists of 129 carbon atoms, 223 hydrogen atoms, three nitrogen atoms, and 54 oxygen atoms. In 1994, scientists managed to re-create this enormous molecule, partly with the help of the Suzuki reaction.

Very challenging creations such as palytoxin force chemists to fine-tune their tools. Furthermore, it is important to re-create, for purposes of research, naturally occurring molecules in test tubes. When scientists find a new molecule they use different chemical methods to establish how its atoms are positioned in relation to eachother. However, the only way to verify this structure is by re-creating the molecule artificially and making a comparison.
A tool in the search for new medicine …
As is evident from the introductory tale about the marine sponge, palladium-catalyzed cross coupling is an important tool in the search for new drugs. Today, scientists around the world use the oceans as a large pharmacy. They have isolated thousands of substances from organisms living in the sea, and these substances have inspired further scientific progress. Besides discodermolide, palladium-catalyzed cross coupling has also helped chemists to artificially synthesize diazonamide A, a substance originating in a Philippine ascidian. In laboratory experiments, diazonamide A has proven effective against colon cancer cells. Another example is dragmacidin F, which has been isolated from a sponge living off the coast of Italy. Preliminary laboratory testing shows that dragmacidin F affects both the herpes virus and HIV.
Chemists also use palladium-catalyzed cross coupling to modify naturally occurring medicinal substances to increase their efficacy. An example of this is vancomycin, an antibiotic that was first isolated in the 1950s from a soil sample taken in the jungles of Borneo. Today, vancomycin is used against MRSA and entero-cocci, bacteria that have become resistant to our more commonly used antibiotics. Both these bacteria are usually quite harmless, but they can infect wounds as well as cause problems after transplantations. Because of this alarming development, scientists are attempting to modify vancomycin so that it is also effective against vancomycin-resistant strains. Using palladium-catalyzed cross coupling they have created variants of vancomycin that work on resistant bacteria.
… and thinner computer screens
The electronics industry is also making use of palladium-catalyzed cross coupling, for example when it comes to finding better light sources for diodes. Organic light emitting diodes, OLEDs, consist of organic molecules that emit light. They are used in the electronics industry in the production of extremely thin monitors, just a few millimetres thick. Scientists have used palladium-catalyzed cross coupling in order to optimize the blue light in the OLEDs.
Never-ending progress
As the Heck reaction, the Suzuki reaction and the Negishi reaction are all of vital importance for the creation of ever more complex chemicals, other chemists have optimized and altered them. One such alteration is linked to this year’s Nobel Prize in Physics. In spring 2010, scientists announced that they had attached palladium atoms to graphene, and the resulting solid material was used to carry out the Suzuki reaction in water.
The palladium-catalyzed cross-coupling reaction is still being developed, even though it is now more than 40 years since Richard Heck first began experimenting in his Delaware laboratory. The discoveries of Richard Heck, Ei-ichi Negishi and Akira Suzuki, are already of great importance to humanity. However, taking into account the developments currently being made in laboratories worldwide, their reactions are likely to become even more important in the future.
Links and further reading
Scientific articles
Heck, R. F. and Nolley, J. P. (1972) J. Org. Chem. 37, p. 2320.
Negishi, E.-I., King, A. O. and Okukado, N. (1977) J. Org. Chem. 42, p. 1821.
Miyaura, N. and Suzuki, A. (1979), J. Chem. Soc. Chem. Commun., p 866.
Review articles
Negishi, E. (1999) A profile of Professor Richard F. Heck Discovery of the Heck reaction. Journal of Organometallic Chemistry 576, p. XV-XVI.
Rouhi, M. (2004) Chem. & Eng. News, 82 (36), Sept. 6, p. 49–58. [Article about Suzuki.]
de Meijere, A. and Diederich, F. (Eds.) (2004) Metal-Catalyzed Cross-Coupling Reactions, vol. 1 and 2, Wiley-VCH, Weinheim. pp. 916.
Buchwald, S. L. (Ed.) (2008) Accounts of Chemical Research, Vol. 41, Nov. 11, p. 1439–1564. [Special issue on Cross Coupling.]
The Laureates
RICHARD F. HECK
Quezon City
Philippines
American citizen. Born 1931 in Springfield, MA, USA.
Ph.D. 1954 from University of California Los Angeles (UCLA), CA, USA.
Willis F. Harrington Professor Emeritus at University of Delaware, Newark, DE, USA.
EI-ICHI NEGISHI
H.C. Brown Laboratories of Chemistry
Purdue University
560 Oval Drive
West Lafayette, IN 47907-2084
USA
www.chem.purdue.edu/negishi/index.htm
Japanese citizen. Born 1935 in Changchun, China. Ph.D.
1963 from University of Pennsylvania, Philadelphia, PA, USA.
Herbert C. Brown Distinguished Professor of Chemistry at Purdue University, West Lafayette, IN, USA.
AKIRA SUZUKI
Graduate School of Engineering Hokkaido University
Kita 13, Nishi 8 Kita-ku,
Sapporo 060-8628
Japan
Japanese citizen. Born 1930 in Mukawa, Japan.
Ph.D. 1959, Distinguished Professor Emeritus, both at Hokkaido University, Sapporo Japan.
Science Editors: Jan-Erling Bäckvall, Lars Thelander, The Nobel Committee for Chemistry
Text by Ann Fernholm
Illustrations by Airi Iliste
Editor: Annika Moberg
© The Royal Swedish Academy of Sciences
Nobel Prizes and laureates
Six prizes were awarded for achievements that have conferred the greatest benefit to humankind. The 12 laureates' work and discoveries range from proteins' structures and machine learning to fighting for a world free of nuclear weapons.
See them all presented here.