Popular information
Information for the public:
Cells and sensibility (pdf)
Populärvetenskaplig information:
Ett sinne för celler (pdf)

The Nobel Prize in Chemistry 2012
In our eyes, noses and mouths, we have sensors for light, odours and flavours. Within the body, cells have similar sensors for hormones and signalling substances, such as adrenalin, serotonin, histamine and dopamine. As life evolved, cells have repeatedly used the same basic mechanism for reading their environment: G-protein-coupled receptors. But they remained hidden from researchers for a long time.
Cells and sensibility
You have been working far too late. The moon lights up the sky as you walk home from the isolated bus stop. Suddenly, you hear footsteps behind you. They approach rapidly. “Nothing to worry about”, you try to tell yourself, “just another poor employee driven too hard at work.” But a creepy feeling takes hold. Someone is really after you …
You flee towards your home. As you unlock the front door, your entire body is shaking, your heart is pounding and you are gasping for breath.
In the same moment that your eye registered the approaching silhouette, your entire body switched into flight mode (figure 1). Nerve signals from the brain sent an initial warning to the body. The pituitary gland released hormones to the bloodstream that awakened the adrenal gland. It started pumping out cortisol, adrenalin and noradrenalin that issued a second warning: it’s time to flee! Fat cells, muscle cells, liver, heart, lungs and blood vessels all reacted immediately. The blood got flooded with sugar and fat, the bronchi expanded and your heart rate increased – all so that your muscles could get more energy and oxygen. The aim is to make you run as fast as you can in order to save your life.
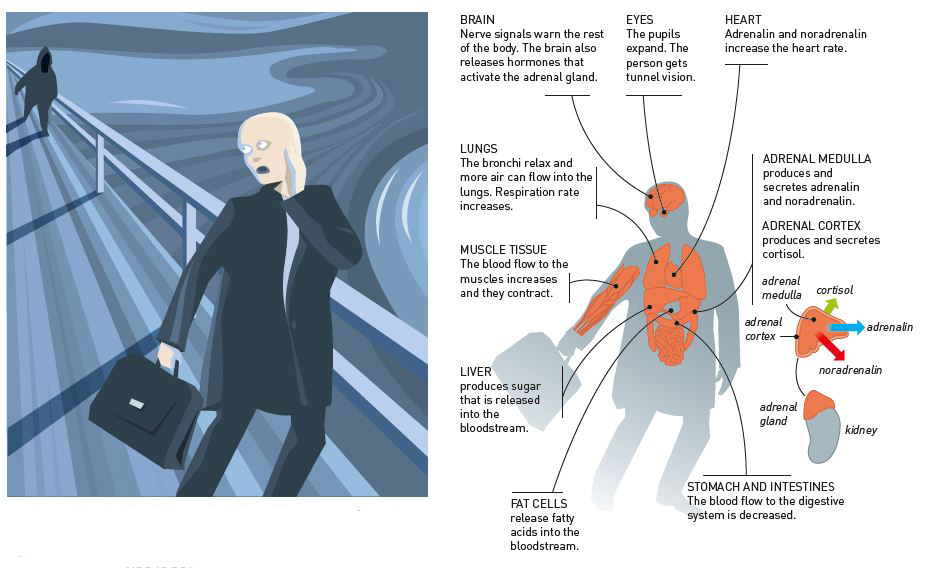
In a human, tens of thousands of billions of cells interact. Most of them have developed distinct roles. Some store fat; others register visual impressions, produce hormones or build up muscle tissue. In order for us to function, it is crucial that our cells work in unison, that they can sense their environment and know what is going on around them. For this, they need sensors.
Sensors on the cell surface are called receptors. Robert J. Lefkowitz and Brian K. Kobilka are awarded the 2012 Nobel Prize in Chemistry for having mapped how a family of receptors called G-protein-coupled receptors (GPCRs) work. In this family, we find receptors for adrenalin (also known as epinephrine), dopamine, serotonin, light, flavour and odour. Most physiological processes depend on GPCRs. Around half of all medications act through these receptors, among them beta blockers, antihistamines and various kinds of psychiatric medications.
Knowledge about GPCRs is thus of the greatest benefit to mankind. However, these receptors eluded scientists for a long time.
The receptor – an elusive enigma
At the end of the 19th Century, when scientists began experimenting with adrenalin’s effects on the body, they discovered that it makes the heart rate and blood pressure increase and also relax the pupils. Because they suspected that adrenalin worked via nerves in the body, they paralyzed the nervous system of laboratory animals. However, the effect of the adrenalin still manifested itself. Their conclusion: cells must have some kind of receptor that enables them to sense chemical substances – hormones, poisons and drugs – in their environment.
But when researchers attempted to find these receptors, they hit the wall. They wanted to understand what the receptors look like and how they convey signals to the cell. The adrenalin was administered to the outside of the cell, and this led to changes in its metabolism that they could measure inside the cell. Each cell has a wall: a membrane of fat molecules that separates it from its environment. How did the signal get through the wall? How could the inside of the cell know what was happening on the outside?
The receptors remained unidentified for decades. Despite this, scientists managed to develop drugs that specifically have their effect through one of these receptors. In the 1940s, the American scientist Raymond Ahlquist examined how different organs react to various adrenalin-like substances. His work led him to conclude that there must be two different types of receptors for adrenalin: one that primarily makes smooth muscle cells in blood vessels contract, and another that primarily stimulates the heart. He called the receptors alpha and beta. Soon after this, scientists developed the first beta blockers, which are currently some of our most frequently used heart medicines.
Such drugs undoubtedly produced effects in the cells, but how they did so remained a mystery. We now know why the receptors were so difficult to find: they are relatively few in number and they also are mostly encapsulated within the wall of the cell. After a couple of decades, even Ahlquist began to feel lost in his theory about the two distinct receptors. He writes: “To me they are an abstract concept conceived to explain observed responses of tissues produced by chemicals of various structures.”
It is here at the end of the 1960s that Robert Lefkowitz, one of this year’s Nobel Laureates, enters the history of these receptors.
Luring receptors out of their hiding places
The young top student has his mind set on becoming a cardiologist. However, he graduates at the height of the Vietnam War, and he does his military service in the US Public Health Service at a federal research institution, the National Institutes of Health. There he is presented with a grand challenge: finding the receptors.
Lefkowitz’s supervisor already has a plan. He proposes attaching radioactive iodine to a hormone. Then, as the hormone binds to the surface of a cell, the radiation from the iodine should make it possible to track the receptor. Furthermore, in order to strengthen his case, Lefkowitz would have to show that the hormone’s coupling to the cell’s outside actually triggers a process known to take place on the inside of the cell. If he could succeed in this, no one could doubt that he had in fact discovered a biologically functioning receptor.
Lefkowitz begins working with adrenocorticotropic hormone, which stimulates the production of adrenalin in the adrenal gland. But nothing seems to work. A year passes, yet he makes no progress, and Lefkowitz, who really was not that keen on doing research in the first place, begins to despair. He continues his research but dreams of becoming a medical doctor.
As the project enters its second year, Lefkowitz finally makes some progress. In 1970, he publishes articles in two prestigious journals, Proceedings of the National Academy of Sciences (PNAS) and Science, where he outlines the discovery of an active receptor. The achievement makes him appreciate the thrill of doing research, and eventually he is recruited to Duke University in North Carolina. It’s not that he is particularly keen on moving there, but they make him an offer that he just cannot refuse.
In brand new laboratories, Lefkowitz forms his own research team. Although it looks like he will never became a cardiologist, he still wants to work with heart disease. So, he begins focusing on receptors for adrenalin and noradrenalin, so-called adrenergic receptors. Using radioactively tagged substances, including beta blockers, his research group examines how these receptors work. And after fine-tuning their toolkits, they manage with great skill to extract a series of receptors from biological tissue.
Meanwhile, the knowledge about what happens inside cells has been growing. Researchers have found what they call G-proteins (Nobel Prize in Physiology or Medicine 1994) that are activated by a signal from the receptor. The G-protein, in turn, triggers a chain of reactions that alters the metabolism of the cell. By the beginning of the 1980s, scientists are starting to gain an understanding of the process by which signals are transmitted from the outside of the cell to its inside (figure 2).
The gene – a key to new insights
In the 1980s, Lefkowitz decides that his research group should try to find the gene that codes for the beta receptor. This decision would prove to be crucial to this year’s Nobel Prize. A gene is similar to a blueprint. It contains a code that is read by the cell when it joins amino acids to create a protein, for instance, a receptor. The idea was that if the research group could isolate the gene and read the blueprint for the beta receptor, they could get clues as to how the receptor works.
At about the same time, Lefkowitz hires a young doctor, Brian Kobilka. His fascination with adrenergic receptors was born of his experience in hospital intensive care. A shot of epinephrine could be the difference between life and death. The hormone opens up a swollen respiratory system and speeds up the heart rate. Kobilka wanted to study the power of epinephrine in its smallest molecular detail, and so approached Lefkowitz and his team of researchers.
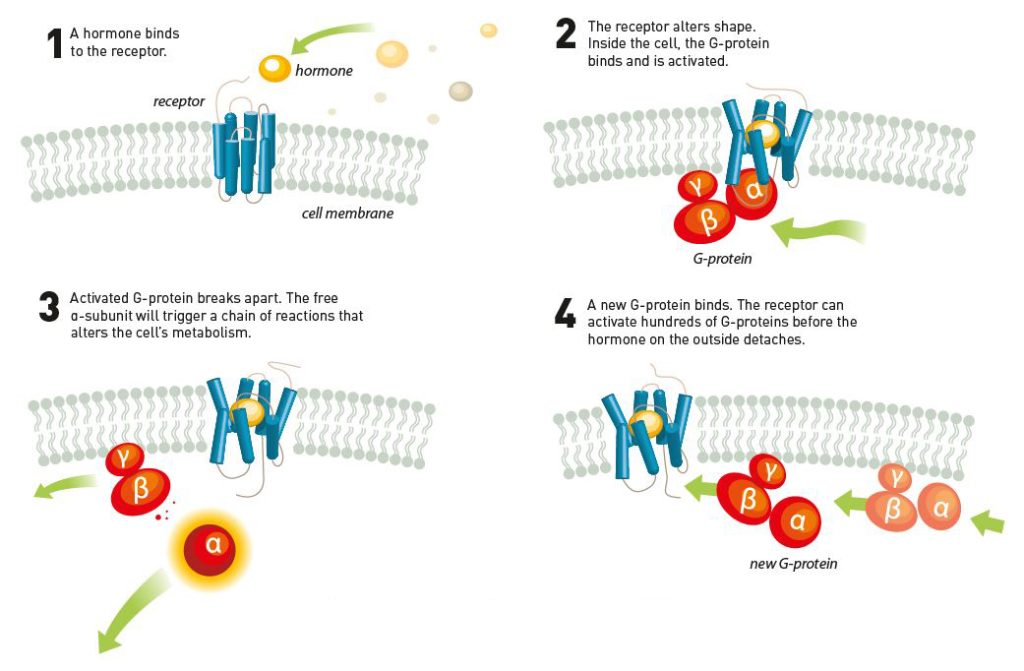
Kobilka engages in the hunt for the gene. However, during the 1980s, trying to find a particular gene in the body’s enormous genome is a bit like trying to find a needle in a haystack; the technically challenging project is slow to advance. However, Kobilka has an ingenious idea that makes it possible to isolate the gene. With great anticipation, the researchers begin to analyze its code; it reveals that the receptor consists of seven long and fatty (hydrophobic) spiral strings – so-called helices (figure 3). This tells the scientists that the receptor probably winds its way back and forth through the cell wall seven times.
Seven times. This was the same number of strings and same spiral shape as a different receptor that already had been found elsewhere in the body: the light receptor rhodopsin in the retina of the eye. An idea is born: could these two receptors be related, even though they have completely different functions?
Robert Lefkowitz later described this as a “real eureka moment”. He knew that both adrenergic receptors and rhodopsin interact with G-proteins on the inside of the cell. He also knew of about 30 other receptors that work via G-proteins. The conclusion: there has to be a complete family of receptors that look alike and function in the same manner!
Since this groundbreaking discovery, the puzzle has been assembled bit by bit, and scientists now have detailed knowledge about GPCRs – how they work and how they are regulated at the molecular level. Lefkowitz and Kobilka have been at the forefront of this entire scientific journey, and last year, in 2011, Kobilka and his team of researchers reported a finding that put the crown atop their work.
Imaging adrenalin effects
After successfully having isolating the gene, Brian Kobilka transferred to Stanford University School of Medicine in California. There he set out to create an image of the receptor – an unattainable goal in the opinion of most of the scientific community – and for Kobilka, it would become a long journey.
Imaging a protein is a process involving many complicated steps. Proteins are too tiny to be distinguished in regular microscopes. Therefore, scientists use a method called X-ray crystallography. They begin with producing a crystal, where proteins are tightly packed in a symmetrical pattern, like water molecules packed in an ice crystal or carbon in a diamond. Researchers then shoot X-rays through the protein crystal. When the rays hit the proteins, they scatter. From the pattern of this diffraction, scientists can tell what the proteins look like at the atomic level.
The first image of a crystal structure of a protein was produced in the 1950s. Since then, scientists have X-rayed and imaged thousands of proteins. However, the majority of them have been water-soluble, which facilitates the crystallization process. Fewer researchers have managed to image proteins located in the fatty membrane of the cell. In water, such proteins dissolve just as poorly as oil, and they are prone to form fatty lumps. Furthermore, GPCRs are by nature very mobile (they transmit signals by moving), but inside a crystal they have to remain almost completely still. Getting them to crystallize is therefore a considerable challenge.
It took Kobilka over two decades to find a solution to all these problems. But thanks to determination, creativity and molecular biology sleight of hand, Kobilka and his research group finally achieved their ultimate goal in 2011: they got an image of the receptor at the very moment when it transfers the signal from the hormone on the outside of the cell to the G-protein on the inside of the cell (figure 3).

The image, published in Nature, reveals new details about GPCRs, for instance, what the activated receptor looks like when it opens up a void where the G-protein likes to bind (figure 4). Such knowledge will be very useful in the future for the development of new pharmaceutical drugs.
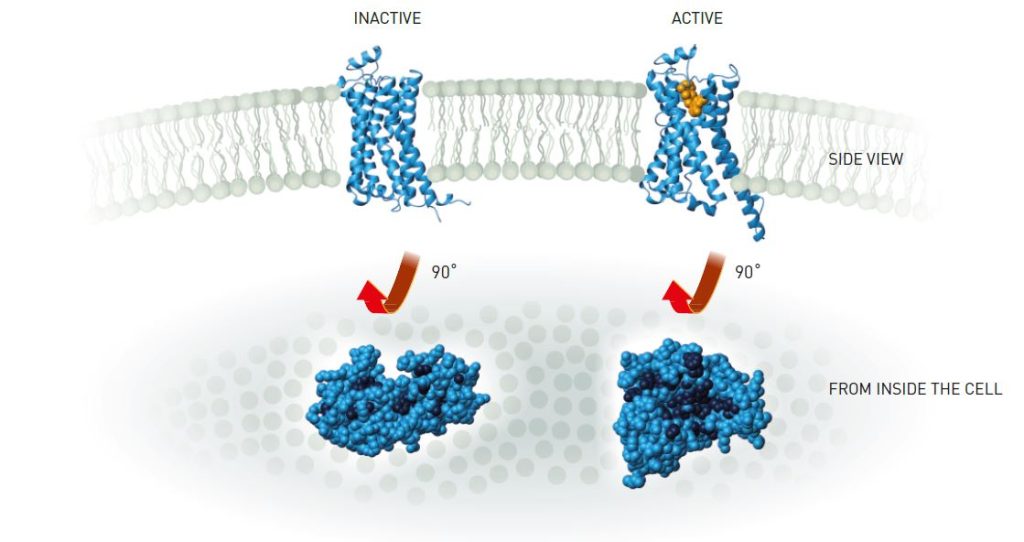
Life needs flexibility
The mapping of the human genome has revealed close to a thousand genes that code for GPCRs. About half of those receptors receive odours and are part of the olfactory system. A third of them are receptors for hormones and signalling substances, such as dopamine, serotonin, prostaglandin, glucagon and histamine. Some receptors capture the light that hits the eye, while others are located on the tongue and give us our sense of taste. Over one hundred receptors still present challenges to scientists, as their purposes have yet to be figured out.
Besides discovering the receptors’ many variations, researchers, with Lefkowitz and Kobilka in the lead, have found that they are multifunctional; a single receptor can recognize several different hormones on the outside of the cell. Moreover, on the inside, they not only interact with G-proteins, but also, for instance, with proteins called arrestins. The realization that these receptors are not always coupled to G-proteins has led scientists to begin referring to them as seven-transmembrane receptors (7TM), after the seven spiral-shaped strings that wind their way through the cell wall.
The receptors’ number and flexibility enable the fine-tuned regulation of cells that life requires. Let us return to the flight scene at the bus stop. When the blood is filled with adrenalin, different tissues react in different ways. The blood flow to the digestive organs diminishes; meanwhile, the flow to the muscles increases. Adrenalin’s different effects depend on there being at least nine different receptors for this hormone in our bodies. Some receptors trigger cell activity, while others have a tranquilizing effect.
So, next time you get scared, savour the taste of good food, or simply gaze at the stars in the sky, give a thought to your G-protein-coupled receptors. Without them, your cells would be striving for conflicting goals, and chaos would reign in your body.
Links and further reading
Articles
Buchen, L. (2011) Cell signalling caught in the act, Nature 475:273–274.
Buchen, L. (2011) It’s all about the structure, Nature 476: 387–390.
Snyderman, R. (2011) Introduction of Robert J. Lefkowitz, J. Clin. Invest. 121(10):4192–4200.
Williams, R. (2010) Robert Lefkowitz: Godfather of G Protein-Coupled Receptors, Circ. Res. 106:812–814.
Lectures (video)
Lefkowitz, R. J. (2010) Part 1 Seven Transmembrane Receptors, http://www.youtube.com/watch?v=gPyo7k9E_-w
Lefkowitz, R. J. (2010) Part 2 Beta-arrestins, http://www.youtube.com/watch?v=IP4zsbemW8I
The Laureates
ROBERT J. LEFKOWITZ
U.S. citizen. Born 1943 in New York, NY, USA. M.D. 1966 from Columbia University, New York, NY, USA. Investigator, Howard Hughes Medical Institute. James B. Duke Professor of Medicine, and Professor of Biochemistry, Duke University Medical Center, Durham, NC, USA.
www.lefkolab.org
BRIAN K. KOBILKA
U.S. citizen. Born 1955 in Little Falls, MN, USA. M.D. 1981 from Yale University School of Medicine, New Haven, CT, USA. Professor, Chair of molecular and cellular physiology och Hélène Irwin Fagan Chair in Cardiology, Stanford University School of Medicine, Stanford, CA, USA.
http://med.stanford.edu/kobilkalab
Science Editors: Sara Snogerup Linse and Sven Lidin, the Nobel Committee for Chemistry
Text by Ann Fernholm
Illustrations, unless otherwise indicated: © Johan Jarnestad/The Royal Swedish Academy of Sciences
Editor: Ann Fernholm
© The Royal Swedish Academy of Sciences
Nobel Prizes and laureates
See them all presented here.