Richard Henderson
Biographical

Early years
I was born in Edinburgh, Scotland, on 19th July 1945. My mother Grace Goldie, after two weeks convalescence, took me back on the train to Berwick-upon-Tweed, England, to re-join my father John Henderson, who was a baker at Bryson’s in Berwick. My mother was born in Edinburgh and my father in Tadcaster, North Yorkshire. They met in Edinburgh and started their married life in Berwick, where my father had been brought up. We lived in several rented flats in Berwick before moving when I was 3 years old to a council house across the river in Tweedmouth, where my father kept pigeons and then budgerigars. My mother persuaded Tweedmouth primary school to let me start early when I was four years old. I have no idea why she was so keen to get me out of the house. Just before my sixth birthday, my parents moved to the small rural village of Newcastleton, 2 miles north of the border between Scotland and England. My father was one of four bakers working in the local bakery, Oliver’s, graduating from baking bread to cakes. I attended Newcastleton primary school for 5 years (aged 6 to 10). Towards the end of primary school, the four most academic pupils in our class of 19 (Figure 1) were selected to attend Hawick High School, which is about 20 miles north of Newcastleton and was at that time accessible by a 45-minute morning and evening journey by steam train. We would set off at 8am every morning and get back at 5pm each evening. The total travel time of over an hour per day meant we were able to complete any homework during the train journeys. At Hawick, the classes were “streamed” by academic ability, with the entry year having 13 classes of about 35 pupils per class. The four of us from Newcastleton were in the most academic A stream (see Figure 2) and thrilled to be taught Chaucer, Shakespeare, Latin and French as well as Science, Mathematics and my favourite Metalwork. I also remember being delighted to find that mathematics lessons were subdivided into algebra, geometry and trigonometry.
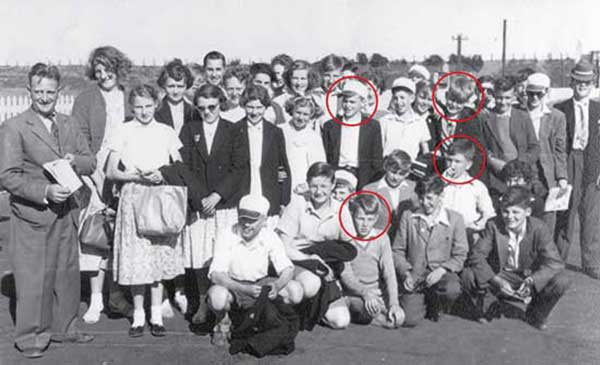
Figure 1. Primary school photo of an outing to Silloth, a seaside resort in Cumberland across the border in England, when I was 10 years old. The photo shows pupils from two classes, ours and the one from the year ahead. My three classmates Robert Davidson and Foster Harkness (rear left to right), and Maurice Carruthers (front right), who subsequently travelled by train to Hawick High School every day, are circled, with Richard Henderson in the centre of the front row.
My great aunt, who owned a corner shop in Edinburgh, sent me every week copies of all the children’s comics that were published in the 1950s, so I did read but definitely not literature. Although my mother tried to persuade me to read when I was young, I did not succeed in completing any novel until the compulsory school English syllabus forced me to read Walter Scott’s “Heart of Midlothian”, which I managed by reading 12 pages each night. When my paternal grandmother died, she left me a set of Arthur Mee’s “Children’s Encyclopedia”, which kept me occupied, especially on “Things to make and do”. Most children left school aged 15 at that time, so in our fifth and sixth form examinations, then called Highers and Lowers in Scotland, the entry year of about 400 pupils had dropped to about 55. For my final two years, at age 15 in Hawick and 16 in Edinburgh, my parents received a £50 family allowance to encourage them to encourage me to continue into higher level education.

Figure 2. Hawick High School class 1A photo 1956. Three people from Newcastleton, circled left to right, are Richard Henderson, Robert Davidson and Maurice Carruthers.
When I was 15, the bakery where my father worked in Newcastleton ran into financial troubles. My father resigned and took a new job in Edinburgh about 3 months before I would sit my first national school examinations in June. My mother and younger brother Ross moved with him to Edinburgh, but it was decided that it would be best if I stayed on for 3 months with our next-door neighbours in Newcastleton, the Zurbriggens, so that I could sit the examinations without having to move to a new school. For my final (6th) year of High School, I moved to Boroughmuir in Edinburgh, following a recommendation by John Low, the headmaster at Hawick.
During that final school year at Boroughmuir, we were all asked whether anyone would like to apply to Oxford or Cambridge University, for which extra lessons geared to their entrance exams would be given, but only one Latin and Greek scholar decided to apply, unsuccessfully. Everyone else decided to apply to Edinburgh University, so when I started a Physics degree course at Edinburgh, I had four or five classmates from Boroughmuir and a similar number from Hawick. Our fourth and final year in Physics at Edinburgh University had a class of 45, including 4 others from Boroughmuir and one other from Hawick. Our final year Physics class photo is shown in Figure 3. The large representation from Boroughmuir arose from the enthusiastic teaching of our Boroughmuir physics teacher Bill Cow, or “Bilko”. Bill once played a recording to the 6th form physics class that he had made of a lecture by Dr Jack Dainty, Reader in Biophysics at Edinburgh University, in which he talked about his work on ion fluxes in Nitella, algae with giant cells. Bill’s view was that biophysics was an important developing area in the future of physics. Although it did not make a deep impact on my thinking at the time, it is possible that my later decision to follow a career in biophysics derived from this initial exposure to Bill Cow’s enthusiasm.
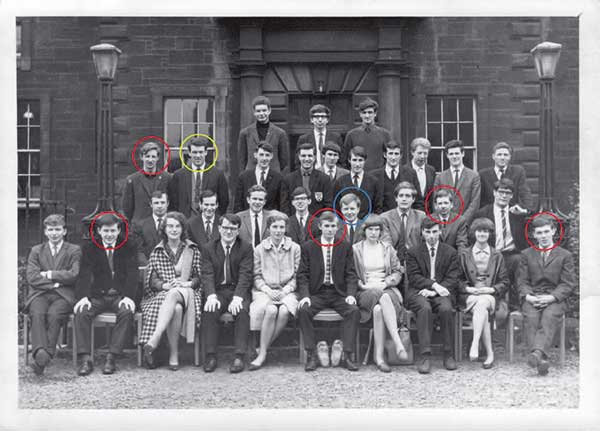
Figure 3. Final year Physics IV class photo in 1966, with those from Boroughmuir, David Hogg, Andrew White, Harry Dooley, Brian Renwick and Richard Henderson (left to right) circled in red. Brian Mitchell from Hawick (yellow), and Craig Mackay who also came to Cambridge and became an astronomer (blue) are also marked. Andrew and Brian were the co-owners of our first, very old car.
My mother, Grace, and both grandmothers were my strongest supporters; my father and grandfather were very busy working and often tired after working all day. My mother had to leave school at 14 to help earn money for her family (her father had been unemployed from 1930–1938 in the Depression), but had really wanted to continue in school, so my higher education allowed her vicariously to fulfil some of her aspirations. She was very supportive and delighted when I did well academically.
The following are some brief memories of the four schools I attended.
1. My first school was Tweedmouth West First School (at age 4–5), which was a 10-minute walk from our house. I can remember even then being fascinated by numbers, and less interested in literary topics. There was a strong emphasis on learning arithmetic skills, and I can remember reciting the “times tables” while walking to school. My paternal grandmother, Jinny Henderson, lived next door to the school, and a great aunt on my father’s side a few doors farther down the same street, so occasionally I would visit my grandmother for lemonade and a biscuit on my way home.
2. When we moved to Scotland, I attended Newcastleton Primary School. Newcastleton is a small village midway between the Scottish and English towns of Hawick and Carlisle, each about 20 miles away in opposite directions. Its population then was about 800, but this has since dwindled to about 600. The two teachers I remember were Miss Russell and Mrs Fleming (when I was aged 6–10). During the summer when we moved, my mother realised that the Scottish schools were ahead of the English schools in their syllabus, so I was set some exercises by Miss Russell during the summer holidays, so that I could catch up. One difference was that my new classmates had all graduated to writing with “joined up” letters, whereas in Tweedmouth everyone was still writing using separate “printed” lettering. In those days also, the UK currency consisted of pounds, shillings and pence. So, when our class was set some problems involving the long-division of money, which my new schoolmates had already been taught, I remember having no trouble completing the task from first principles and getting the correct answer. However, I was told that my procedure was not correctly laid out and that I should not make up my own method. Towards the end of primary school, our class was subjected to a series of tests or qualifying exams that were used to decide on the type of secondary school education each pupil would be offered. Scotland had introduced universal free education up to age 15 in 1945, in a parallel reform to follow the 1944 Butler Education Act in England and Wales. I realised much later that the tests were the Scottish equivalent of the 11-plus exam, although, having started school earlier, I was only 10 years old at the time. The outcome of this testing was that 4 of us from a class of 19 were sent off to Hawick High School, leaving the remainder of the class to continue for another 3 years of secondary education in Newcastleton, which had a separate wing for older pupils.
3. At Hawick High school, when I was aged 11–15, the teachers I remember were “Jeemie” Allen, an excellent maths teacher and dahlia fancier, and Bill McLaren, who taught us for gym and rugby. One of my Hawick classmates, Myra Thomson, wrote to me after the 2017 Nobel Prizes were announced to remind me of the occasion when I was told off by Jeemie for simply writing down the answer to a maths question without bothering to write out the working. I remember being quite surprised when I was 14 to receive a school book prize, valued at 5 shillings, for being placed 3rd in maths, 3rd in science and 3rd equal in geography, having in earlier years always been nearer the bottom of the class. I chose a paperback book called “The Cockleshell Heroes” about a daring kayak raid to sink some German battleships in the French river port of La Rochelle during the second world war. This may have led to my later enthusiasm for kayaking. Although I gradually worked my way up in maths and science at Hawick, my language skills were always poor, and I was the only one in the class to fail the “Lower” in French. In Hawick, there was a shop I visited in the school lunch hour along with one or two friends, which sold ex-WD (War Department) electronics, so we would acquire very inexpensive components and build our own valve radios.
4. At the end of my 5th year of secondary school, the headmaster at Hawick recommended three schools in Edinburgh for my final (6th) year of secondary school, namely Royal High, George Heriot’s or Boroughmuir. The first two had (nominal) school fees whereas Boroughmuir was free, so we chose Boroughmuir Secondary School. My final year of school, at age 16, was spent at Boroughmuir, with Bill Cow, an excellent Physics teacher, and Dr Young our chemistry teacher, who had a Ph.D. and had worked on explosives during the war. Since we had already finished our science “Highers” examinations at the end of the 5th form, the 6th form science had no special curriculum. One difference between Hawick and Boroughmuir was that the sciences were taught in an integrated class by a single teacher at Hawick, whereas there were separate physics, chemistry and biology classes at Boroughmuir. Boroughmuir also had selective entry, so that the overall academic level and teaching standards were higher than at Hawick, which was an all-inclusive comprehensive school. A few of us expressed an interest in biology and studied some plant biology in our free periods. Dr Young also allowed us to choose any chemistry experiment we wanted to do on Friday afternoons, when we had a double period of Chemistry. One member of our class decided to take advantage of our teacher’s wartime experiences to test a different explosive each week, so every Friday at around 3.30pm we all had to crouch down below the bench while that week’s test explosive was ignited. Only once was the explosion strong enough to leave marks on the walls. My abysmal performance in French was rectified by having tuition in the 6th year in a class that had only two pupils. I had failed Lower French at Hawick and my classmate had failed Higher French at Boroughmuir. After an entire year of individual tuition, I scraped through the French exam with a bare pass and was thus able to meet the entrance requirements for the University of Edinburgh.
In my academic education, I thus benefitted greatly from the post-war education reforms, which opened new opportunities for working class children and brought in free secondary school education for all. At one stage aged 13, I tried to drop French in high school, but our headmaster called me into his office and told me that I should not do that if I wanted to keep open the option of going to University, because a language at O-level (Scottish Lower) was an entry requirement. I don’t think I even knew of the existence of Universities at that point.
University of Edinburgh (age 17–20)
I pursued a B.Sc. in “Natural Philosophy” which was the traditional name for Physics. This consisted of 4 years of physics, maths and mathematical physics. Peter Higgs was our mathematical physics lecturer in 1962–64, at just about the time he was writing his famous paper predicting what came to be known as the “Higgs boson”. I was delighted finally to be allowed to focus entirely on the subjects I found most interesting.
During my undergraduate years, I took many jobs during the Christmas, Easter and Summer breaks, partly to earn enough money to pay for running a car, and partly to get direct experience of different working environments. With two school friends, we bought a car (£10 each) when we were 17. The car, a 15-year-old Morris 8 Series E, was very unreliable, so the three of us learned a lot about car engines, clutches, gearboxes, back-axles and half-shafts, since they all seemed to need frequent repair or replacement, mostly from scrapyards. Although only one of us had passed his driving test, he taught the other two. During the three summers from 1963 to 1965, I worked in the technical drawing office at the electrical engineering company Ferranti designing a slide projector, at the UK Atomic Energy Authority (UKAEA) at AWRE Aldermaston in a small group evaluating lithium-drifted germanium detectors for gamma rays, and with Dr John Muir in the Physics Department on a summer project using microwaves to analyse the dielectric properties of kaolinite clay, with support from a Carnegie Trust Vacation Scholarship. Each of these three positions exposed me to different cultures. In the company, it was very hierarchical: the man in charge of the drawing office did not cope well with the more competent students. At UKAEA, a science campus operating as part of the civil service, there were many brilliant scientists who were a pleasure to work alongside, but their research was part of bigger projects, so their enthusiasm was muted. In contrast, those carrying out research in the university were enthusiastic, highly motivated and clearly excited about their work. I therefore decided that an academic research career would be my best option.
Decision to choose biophysics for graduate research
During my final year as an undergraduate, I spent a long time trying to decide which of the many exciting directions that physics was taking would be most interesting for me in a future research career. I can remember considering fusion research which promised to provide unlimited power generation, solid state physics which has transformed our lives through development of a multitude of semiconductor devices, high energy particle physics which has led to a deep understanding of nuclear structure, or astrophysics which has transformed our understanding of the universe from the big bang to black holes, neutron stars and gravitational waves. In the end, I decided that biophysics had great potential in bringing the power of physics to understand biological phenomena. One of the most important factors in making this choice was that I was keen to do individual hands-on research either myself or with one or two close colleagues, rather than to work as part of a large team, which would have been essential for some of the other directions. The final year physics exam in Edinburgh consisted of 6 papers on successive days on different topics ending with a final essay paper with a broader scope, and encouragement to be somewhat light-hearted. I wrote an essay on “Time”, in which I explained that time consisted of the past, present and future. Since the past and present could simply be looked up in a history book or encyclopaedia, only the future was interesting. This then led into a consideration of where physics was heading, with a discussion of the above range of topics and ending up with the conclusion that biophysics had great potential and might offer rewarding opportunities for the individual.
Having decided on biophysics, the question was then where to go for a Ph.D. research project. Since, at the age of 20, I had no desire to do any more studying, attending lectures or sitting exams, this ruled out all American Universities, and on further investigation also ruled out Leeds (R.D. Preston, successor to Astbury) and Norwich, where Jack Dainty had moved to a Professorship, his promotion having been turned down by Edinburgh. Both those biophysics departments had compulsory M.Sc. degrees that took at least a year before they would allow students to pursue research for Ph.D. That left only King’s College London, which I visited in November 1965. I talked with many people at Kings (Randall, Wilkins, Jean Hanson, Jack Lowy, Watson Fuller, Struther Arnott), and eventually wrote back after my return to Edinburgh asking whether I might be allowed to work on surface forces with Dr Anita Bailey. King’s did not offer me a place immediately but said they would let me know next Spring. Having made my plan after a lot of investigation, I thought it might be tactful to go and tell our new Professor, Bill Cochran, who had arrived from Cambridge in 1964 and quickly built up an outstanding solid-state physics group, about my decision to go into biophysics. Without hesitation, he quickly advised me that I should write to his friend Max Perutz at the Medical Research Council (MRC) Laboratory of Molecular Biology in Cambridge (MRC-LMB). Somehow, in spite of all my efforts to talk to many other people in Edinburgh and to contact and visit other places around the UK, I had not managed to identify the MRC-LMB. This was primarily because most research in UK universities at that time was listed in the Science Research Council (SRC) Handbook. The MRC-LMB in Cambridge was listed only in the MRC Handbook, which was not available in the Physics Department. In contrast, King’s College Biophysics was both a Biophysics Department in the University and an MRC Biophysics Unit, so was listed in both handbooks.
I therefore wrote to Max Perutz in January, received a reply in February and visited the MRC-LMB on a Saturday morning in March for the student Open Day, arriving on the overnight train from Edinburgh and returning on the same evening. There were about 20 students visiting, almost all from Cambridge. David Blow gave an informal talk about some of the research. I was also interviewed individually by Max Perutz and John Kendrew. Kendrew simply asked whether I had any questions. I said I was concerned that I had studied no biology or chemistry, only physics and maths, but he said I should not worry: I could easily pick up biology and chemistry as I went along. The laboratory was a hive of activity with more people at work on a Saturday morning at MRC-LMB than in other places I had visited midweek.
On my return to Edinburgh I therefore immediately wrote to say I would be very interested in becoming a Ph.D. student at MRC-LMB. Perutz wrote back two days later accepting me. That year two physics students started as Ph.D. students at MRC-LMB. Peter Gilbert who was a Physics undergraduate from Cambridge was the other. I had also learned from Cochran that another physics student from Edinburgh, Keith Moffat, had gone to MRC-LMB the year before to start a Ph.D. with Max Perutz, so I also wrote to Keith and asked him to tell me a bit more about Cambridge, especially the College system, about which I knew very little. Keith very kindly replied with a 4-page letter giving a thumbnail sketch of the positive and negative aspects of each college. Keith recommended Darwin and Corpus Christi, largely because Corpus had just opened new postgraduate accommodation in 1964 in the George Thomson Building. I spent a year living in the George Thomson building, and later became a fellow at Darwin and an Honorary Fellow at Corpus.
Cambridge (age 21–24)
I carried out research for my Ph.D. at the MRC Laboratory of Molecular Biology in Cambridge (MRC-LMB), with a thesis on “X-ray analysis of chymotrypsin: substrate and inhibitor binding”. With David Blow, my supervisor, and Tom Steitz, who was a Jane Coffin Childs postdoctoral fellow at that time, we worked out the mechanism of action of this enzyme, which was the first serine protease to have its structure determined. It was also the third or fourth protein structure to be determined at atomic resolution, after myoglobin (1959) and lysozyme (1964). The structures of chymotrypsin, ribonuclease and carboxypeptidase were all determined in 1967. By 2018, there were atomic coordinates for 140,000 macromolecular structures deposited in the Protein Data Bank (PDB).
Before I started my postgraduate work in Cambridge, I applied in June 1966 to attend a 2-week Summer School in Molecular Biology and Biophysics in Oxford, which was held to mark the inauguration of the Laboratory of Molecular Biophysics in Oxford, under Professor David Phillips. My application had arrived after all the places had been filled but Max Perutz had also written to Phillips in support of my application. Consequently, I received another letter a few weeks later from Oxford to say that “due to a withdrawal” I could now be offered a place. Thus, after a week or two at MRC-LMB, I spent 2 weeks in Oxford listening to 50 superb lectures by 25 outstanding scientists, including David Phillips, Max Perutz, Fred Sanger, Maurice Wilkins, Aaron Klug and Mark Bretscher. The only lecture for which my notes were almost a blank, save for the word “supercilious”, was by Sydney Brenner. Brenner was arrogant but also very clever, and shared the 2003 Nobel Prize in Physiology or Medicine for his work developing the nematode as a model organism. One of the highlights of life at MRC-LMB in the 1960s was the Saturday morning coffee meeting in the “Molecular Genetics” kitchen where Brenner would entertain six or ten weekend researchers with his wide-ranging, acerbic comments, phenomenal memory and ability to provide an integrated overview of all aspects of molecular biology as it was then.
Having learned only a limited amount of chemistry at school, since the syllabus for Scottish Higher Science at that time stopped with inorganic chemistry, with only the briefest mention of organic chemistry, I attended Cambridge University Part IA organic chemistry in which Peter Sykes was the most memorable lecturer, and also spent one term doing ten afternoon laboratory practicals in synthetic organic chemistry, the most memorable of which was the synthesis of methyl orange.
Towards the end of my Ph.D., I spent some time thinking about what to do next. I had realised by then that MRC-LMB was a superb laboratory with many truly outstanding scientists, and that its success depended on a very deep investigation and understanding of a few very narrow research topics. However, with my own very narrow training in physics and mathematics, I also realised that I would need a much broader grasp of a wider range of problems if I were to be able to choose a productive research topic and research direction, following the philosophy of Peter Medawar’s description of scientific progress as “The Art of the Soluble”. I therefore looked around for a postdoctoral opportunity where I would be able to get a much broader overview of the importance of different research areas across biology. I had been impressed by two scientists at Yale. One was Fred Richards (1925–2009) for his work on the structure and mechanism of ribonuclease, where his broad knowledge and insight had allowed him to provide the definitive explanation of the mechanism of action of the enzyme ribonuclease; although his structural work lagged significantly behind that of another US group at Buffalo led by David Harker, who had determined a better structure for ribonuclease, Richards was much more successful at explaining its importance and in relating structure to mechanism. The second impressive person at Yale was Jui Wang (1921–2016) in the Chemistry Department, who had proposed a hydrolytic mechanism for chymotrypsin that was appealing and showed deep insight into the chemistry of catalysis. I therefore wrote to both enquiring about the possibility of postdoctoral work. Wang replied immediately offering me a place, whereas Richards’ reply, although equally encouraging, did not come for 6 weeks: he was a keen yachtsman and was away sailing. I therefore decided to join Jui Wang and wrote two postdoctoral fellowship applications to the Helen Hay Whitney Foundation (HHWF) and the Jane Coffin Childs (JCC) Memorial Fund. Maclyn McCarty, the chairman of HHWF came to interview Jonathan Greer and me in Cambridge, and we were both subsequently offered HHWF fellowships. Since the HHWF stipend was higher than that of any other postdoctoral fellowship at that time, I accepted their offer, withdrew from JCC, and went to Yale accompanied by my wife Penny and our new-born daughter Jennifer, arriving in New Haven on 20th June 1970. It was Jennifer’s first birthday on the day we arrived at Yale.
Postdoctoral (age 25–27)
I thus ended up as a Helen Hay Whitney Foundation Postdoctoral Fellow at Yale University, in the group of Prof. Jui Wang for 2 years in the Chemistry Department, then spent my third year with Prof. Fred Richards in the Department of Molecular Biophysics and Biochemistry, with a bench in Tom Steitz’ lab. I tried to work on voltage-gated sodium channels in nerve and muscle membranes with the goal of determining the structure, but after 2 years decided that this goal was premature because the methods were inadequate, so then decided to tackle a simpler membrane protein, which was the light-driven proton pump bacteriorhodopsin, which had just been discovered by Walther Stoeckenius in 1971.
When I first arrived at Yale, based on my postdoctoral fellowship application and my own thinking at that time, I had planned to embark on two projects. The first was to label a peptide substrate of chymotrypsin with 13C at its carbonyl carbon and to carry out 13C Nuclear Magnetic Resonance (NMR) analysis to explore the chemical environment in the active site. The second was to choose another enzyme, perhaps slightly more interesting than chymotrypsin, and to purify, crystallise and solve its structure. I had written to David Blow to tell him about my plans and he replied to say that he thought Bob Shulman, then at Bell Labs in Newark, New Jersey might be already trying the 13C experiment, so he recommended that I should contact him. I did contact him and was invited to give a seminar at Bell Labs in 1970, where I met Shulman and Dinshaw Patel, his NMR right-hand man. After my seminar, in which I explained how I planned to go about obtaining an equilibrium concentration of the 13C-labelled enzyme-substrate complex using a high concentration of the substrate leaving group to increase the level of the desired structure by mass action, Shulman said it was a very good idea and they would do it. Since he had much better NMR facilities at Bell Labs than at Yale, I agreed to leave it to them and indeed George Robillard carried out and published the experiment, which provided some interesting insights.
I discussed my second proposed project with Wang. This was to purify and crystallise another enzyme. For this, I had selected NADP reductase from spinach, and purified it with one of Wang’s Ph.D. students, Jim Keirns. We succeeded in producing small pale-yellow crystals of spinach NADP-reductase before discovering that Martha Ludwig, by then at Ann Arbor, Michigan was already making progress on it. After some discussion with Wang, he explained that there were thousands of enzymes and, since I was still a young postdoc, it would be much better to pick a new longer-term problem that would come to fruition in 20 years, rather than aiming to take a small incremental step in a topical field. After a day or two, I realised that this was very good advice, and abandoned my initial plans to work on enzyme mechanisms and enzyme structure.
At that time, there was a lot of enthusiasm to understand the structure of membrane and membrane proteins, so I asked myself what was the most interesting membrane protein and chose voltage-gated sodium channels. Voltage-gated sodium and potassium channels had been at the heart of the 1963 Nobel Prize winning work of Alan Hodgkin and Andrew Huxley, and for someone with a physics background these were very attractive research targets. In addition, Wang had published some theoretical speculations about the mechanism of ion channels, and was keen for someone to look for microwave emissions from ion channels in nerve membranes as they opened and closed during the action potential. We therefore ordered some microwave equipment, and I began synthesizing some small molecules which I hypothesized might bind to and block sodium channel currents in nerves. After synthesizing 3 or 4 compounds, I then spent a week or two looking around at Yale to find someone who could measure nerve action potentials, ending up by making contact and collaborating with Murdoch Ritchie, who was chairman of the Pharmacology Department on the Medical School campus on the other side of New Haven. Although all my compounds had absolutely zero effect on nerve impulse propagation, this initial contact nevertheless led to a fruitful 3-year collaboration with Murdoch Ritchie and two others based in Ritchie’s laboratory, namely David Colquhoun, who was a sabbatical visitor from London, and Gary Strichartz, another postdoctoral fellow.
Our experiments on ion channels that eventually produced some useful insights began with the idea of producing radiolabelled tetrodotoxin using a tritium gas electrical discharge. Prof. Martin Saunders, also in the Yale Chemistry Department, had a moribund basement laboratory that was full of spider webs, but also housed a fume cupboard, vacuum equipment and 10 Curies of pure tritiated water, T2O. After arranging to have the basement lab reactivated, I managed to produce some tritium-labelled tetrodotoxin, purify it using flat-bed electrophoresis, and in collaboration with Ritchie demonstrate specific tetrodotoxin binding to nerves and nerve membranes from a variety of sources. We published a number of interesting papers, but my early steps to extract and purify these volt-age-gated sodium channels (VGSCs) were disappointing, because the channels extracted using either Triton X-100 or deoxycholate detergent, were quite unstable with a lifetime of a few minutes at room temperature or a day at 4ºC. I guessed that it might take another 30 years to solve the stability problem so decided to abandon working on VGSCs and choose instead a simpler membrane protein, with the criterion that it should be stable after detergent solubilisation and available in reasonable quantity. The microwave experiments also failed.
In June 1971, I had attended a meeting in San Francisco of the American Society for Biochemistry and Molecular Biology (ASBMB) and heard a wonderful talk by Walther Stoeckenius describing his discovery of the purple membrane from Halobacterium halobium (subsequently renamed as Halobacterium salinarum) and his finding with Dieter Oesterhelt and Allen Blaurock that it was composed of a two-dimensional crystalline array of a single membrane protein to which the chromophore retinal was bound via a Schiff base in a 1:1 stoichiometry, responsible for its characteristic purple colour. After following the work that Stoeckenius and his colleagues Allen Blaurock and Glen King were doing during the next year or two to try to elucidate the structure of the purple membrane, I felt they were heading completely in the wrong direction. Therefore, in early 1973, I decided it would be an opportune time to try one or two new ideas to solve the structure of bacteriorhodopsin, the single protein in purple membrane. Bacteriorhodopsin fitted perfectly the criteria of being stable and available in large amounts. By chance, Don Engelman, then Assistant Professor at Yale, had worked with Stoeckenius as a postdoc a couple of years earlier and knew him well, so Don and I phoned up Stoeckenius and asked whether he could send us a culture of H. halobium, which he kindly did. Neither of my initial ideas, either to use heavy atom derivatives to determine the phases of the powder pattern rings by multiple isomorphous replacement, or to solubilise bacteriorhodopsin and crystallise it in three dimensions for X-ray crystallography, worked out but these were the two approaches I was pursuing when my 3-year postdoctoral fellowship at Yale came to an end.
Penny and I made many friends at Yale and have kept in touch with them over the subsequent decades. During our years in New Haven, Penny gave birth to our second child Elizabeth, born in January 1971, but she had hydroencephalus at birth and died just over seven months later. Our third child, Alastair, was born on 22 March 1973, a few months before our planned return to the U.K.
Back to Cambridge – 1973 until now
Phase I – bacteriorhodopsin at low resolution
We returned to Cambridge on 20th June 1973 to the MRC-LMB on a 5-year appointment, exactly three years after our departure in 1970 (on an American J-1 visa which had a 3-year limit). Jennifer was now four years old and Alastair three months. During my ultimately fruitless efforts to make any progress in analysing the structure of the purple membrane and bacteriorhodopsin using X-ray powder pattern phasing or three-dimensional crystallisation, I was impressed by a talk that Nigel Unwin gave in October 1973 in the annual MRC-LMB symposium. He spoke about electron microscopy (EM) using a phase plate made from a single thread of spider web silk coated with gold. He was clearly thinking that his images of tobacco mosaic virus contained features that represented the protein structure as well as the negative stain he was using to embed the structure. After his talk, we discussed using EM to study the structure of bacteriorhodopsin in its natural two-dimensional crystalline form without using any heavy metal stain. We worked together very productively for about 18 months, ending up with a low-resolution, three-dimensional structure of the first membrane protein, determined by a novel method. The structure showed seven well-resolved trans-membrane a-helices oriented almost perpendicular to the membrane plane, with the implication that this a-helical architecture might be found in other membrane proteins, as indeed it has. After that early success, I switched my efforts from X-ray diffraction to electron diffraction, and eventually to electron microscopy. Nigel switched from working on viruses using negative stain to working on membrane proteins and two-dimensional or helical crystals without using heavy metals, so our 1973–1975 collaboration had a profound impact on the direction of both of our future scientific careers. We were jointly awarded the 1999 Gregori Aminoff Prize of the Royal Swedish Academy of Sciences. A photograph of us taken around that time is shown in Figure 4.
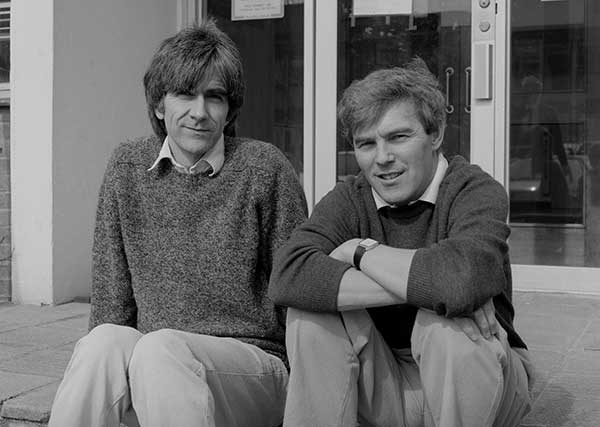
Figure 4. Nigel Unwin and Richard Henderson (left to right) sitting on the steps of the original entrance to the MRC Laboratory of Molecular Biology in the 1990s.
Phase II – bacteriorhodopsin at high resolution
After spending about 7 or 8 years unsuccessfully trying to extend the resolution of our bacteriorhodopsin map from low resolution (7 Å) to high resolution (3 Å), where we expected to resolve the chemistry of the structure (i.e. to see the amino acid side chains and understand the mechanism), I eventually concluded that the methods that our group had been trying (model building, molecular replacement and heavy atom derivatives) were simply not powerful enough, and that we would have to embrace the necessity of recording high resolution electron cryomicroscopy (cryoEM) images. We did this from 1984 until 1990, by visiting and collaborating with a number of other laboratories (EMBL Heidelberg with Jacques Dubochet and Jean Lepault; Fritz Haber Institute Berlin with Fritz Zemlin and Elmar Zeitler; and Berkeley with Ken Downing and Bob Glaeser) as well as trying to improve our in-house EM capabilities in Cambridge. Eventually, the problems of high-resolution cryomicroscopy imaging and computer-based image processing were largely solved and we obtained a high-resolution map in 1990, into which we were able to build a nearly complete atomic model of bacteriorhodopsin. In the end, it was only the second membrane protein structure to be determined at high resolution. The first was Hartmut Michel’s bacterial reaction centre membrane protein complex from R. viridis solved by crystallisation and X-ray crystallography, for which he shared the 1988 Chemistry Nobel Prize with Johann Deisenhofer and Robert Huber. After 1990, Sriram Subramaniam and I did some trapping of intermediates to help work out the mechanism of the bacteriorhodopsin light-driven protein pump. Our bacteriorhodopsin work was essentially completed by 1999.
On a more personal note during this period, Penny and I arranged an amicable divorce in 1988, Jennifer married Richard Morris in 1993, I married Jade Li in 1995, and Alastair married Laura Williams in 1999. The resulting clan including 6 grandchildren all came to Stockholm on 10th December 2017 (Figure 5).
Phase III – single particle cryoEM
From 1995, following publication of a review I wrote on “The potential and limitations of neutrons, electrons and X-rays for atomic resolution microscopy of unstained biological molecules”, I was convinced that the future of cryoEM would involve imaging of single particles embedded in vitreous ice, a specimen preparation method that had been developed by Jacques Dubochet’s group in the 1980s. This “single particle cryoEM” method was potentially very powerful because it did not require the protein of interest to be crystallised nor did it require the use of crystallographic methods, which was what I had worked on exclusively until that point using X-ray and electron diffraction. Single particle electron microscopy had started with the image processing methods of Joachim Frank and Marin van Heel, as well as Owen Saxton and Wolfgang Baumeister, prior to the development of Dubochet’s plunge-freeze method of producing thin films of amorphous ice, but it was the combination of the two that promised to be particularly powerful.
We worked from 1995 until 2013 to analyse the problems and barriers to making progress, and gradually understood and solved them. The most important were the need for brighter sources, better vacuums, more stable cold stages and better detectors. The electron microscope companies, under pressure from users, addressed the first three. Our group in Cambridge, collaborating with another group at Rutherford-Appleton-Laboratory (RAL) near Oxford, worked out how to improve the detectors, and other people developed better computer programs to take advantage of some of the features of the new detectors (Steve Ludtke, Niko Grigorieff, and Sjors Scheres). As a result, almost overnight in early 2013, everyone started to obtain maps with much higher resolution. This was termed the “Resolution Revolution” by Werner Kühlbrandt (Science (2014) 343, 1443). From that point on, there was great enthusiasm from the entire structural biology community and a wider adoption of single particle cryoEM methods, so that now in 2018 cryoEM has become the prime method for many structural biology problems.
Other contributions
I was Joint Head of the Structural Studies Division at MRC-LMB (1986– 1999), Director of MRC-LMB (1996–2006) and a member of the Medical Research Council, which is the governing Board of MRC (2008–2014). Previous Directors were Max Perutz (1962–1979), Sydney Brenner (1979–1986) and Aaron Klug (1986–1996). The current Director is Hugh Pelham (2006–2018). All previous directors at MRC-LMB were also Nobel Prize winners. Probably the most significant achievement that we made during my Directorship was to advocate in 1999 the construction of a new building to house the MRC Laboratory of Molecular Biology in the 21st century, to carefully time the initiative to request funds for the construction to coincide with an upswing in the economic cycle around 2003, and to negotiate the subsequent hurdles for land acquisition and planning permission. This resulted in a superb new 30,000 square metre building, which opened in 2013, is attuned to the needs of modern molecular biology, and has the flexibility to have space reconfigured to parallel the changing needs of research. During my time as Director, Hugh Pelham as Deputy Director took a deep interest in the design and layout of the new building and carried the early planning through to completion during the first half of his Directorship. At the same time, Dr Megan Davies, the Assistant Director from 1996 and Head of the MRC Centre in Cambridge, ensured that our relationships with MRC in London and the local biomedical community in Cambridge were strengthened. An initial invitation soon after I started my Directorship to have dinner with Dr Keith Peters, the Regius Professor of Physik (an old term for Medicine) and Head of the Clinical School, developed into an annual strategic tête-à-tête that helped to keep the interests of MRC-LMB aligned with those of the Clinical School and the NHS Addenbrooke’s Hospital Trust.

Figure 5. Clan gathering in Stockholm. Left to right: Joshua Morris, Jessica Morris, Grace Morris, Rosie Morris, Rachel Henderson, Jade Li, Alastair Henderson, Richard Henderson, Jennifer Morris, Richard Morris, Tom Henderson, Laura Henderson.
Nobel ceremony
My wife, Jade Li, and I spent a wonderful 10 days in Stockholm for the awards of the 2017 Nobel Prizes, with the good fortune to be able to hear the Physics lectures about how gravitational waves were observed for the first time, to meet the Physiology or Medicine laureates who had worked out the molecular basis of circadian rhythms, and also the literature and economics laureates, Kazuo Ishiguro and Richard Thaler. A photograph of our family taken on the stage of Stockholm Concert Hall immediately after the awards is shown in Figure 5.
This autobiography/biography was written at the time of the award and later published in the book series Les Prix Nobel/ Nobel Lectures/The Nobel Prizes. The information is sometimes updated with an addendum submitted by the Laureate.
Nobel Prizes and laureates
Six prizes were awarded for achievements that have conferred the greatest benefit to humankind. The 12 laureates' work and discoveries range from proteins' structures and machine learning to fighting for a world free of nuclear weapons.
See them all presented here.