George P. Smith
Biographical
Early life
I was born March 10, 1941 to Albert Mark Smith II (March 2, 1908 to February 3, 1978) and Jessie Patton Biggs Smith (September 14, 1909 to June 14, 2000). My brother Mark (A. Mark Smith III) was born December 29, 1942 and my sister Helen (now Helen Boyd) was born June 22, 1947.
My father graduated from West Point (the U.S. Military Academy) in 1930, but left the army for civilian life immediately afterward. He had steady employment during the Depression, and he and my mother, who married in 1936, lived in relative prosperity in those years.
The Japanese attack on Pearl Harbor occurred nine months after my birth. My father immediately re-joined the army and continued as a career officer until his retirement in 1965. This meant that our family moved very frequently while Mark, Helen, and I were growing up. By the time I graduated from ninth grade in 1955, I’d attended an extraordinary 11 or 12 schools. Most of the schools were on army bases, where all the kids moved as frequently as we did. That meant that the new kid in school was rapidly assimilated into society, without having to spend a painful year or two as outsider. I learned how to make new friends rapidly, but not how to maintain long-term friendships – a pattern that continues to some degree to the present day.
Mostly my father was posted up and down the East Coast, but during the Korean War he served in Japan from 1951 to 1954. The rest of the family joined him there in April 1952, just as the U.S. occupation was coming to an end.
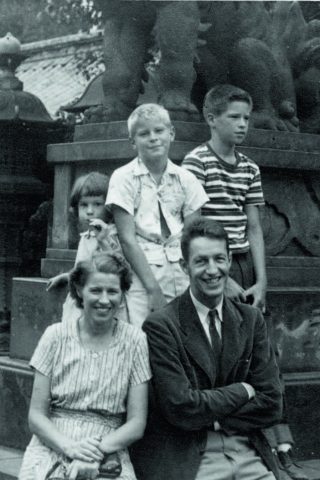
Figure 1. Our family sitting under the stone lion at the Toshogu shrine, Ueno Park, Tokyo, September 7, 1952. I’m the 11-year old dreamer on the right.
My career as herpetologist
In the summer of 1949, our family went on vacation for a few weeks at Penobscot Bay, Maine. The place was overrun with snakes. I ran across two snakes eating the same frog or toad from opposite ends (I don’t know how it turned out). I caught a beautiful green snake and carried it triumphantly by the tail into the parlor, where my mother was hosting a tea party for some proper local ladies. There were some “eeks,” and I was ordered back outside. My herpetology career had begun.
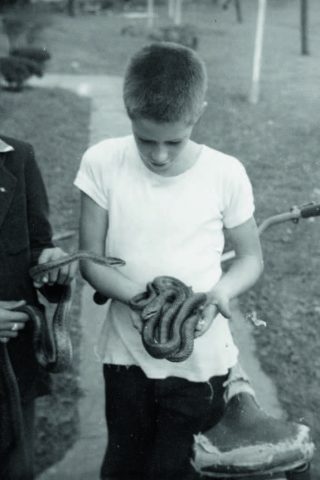
Figure 2. My friend and I have just caught two elegant rat snakes. Washington Heights U.S. military housing complex, Tokyo, summer 1952.
HAM radio
I caught the radio bug while we were still in Japan, and continued through high school. I got my novice license (KN4OWA) around 1956, and built a small rig, which included a classy straight telegraph key I got off an army surplus telegraph set. I never got very proficient in Morse code, however, and dropped out after high school. By that time, I’d learned quite a bit about circuitry, and not a little calculus and analytical geometry (without learning their names), from obsessive study of the Radio Amateur’s Handbook. That’s knowledge that has turned out to be of use in the sequel.
High school
My last three years of schooling were at Philips Academy Andover, a private boarding school in Massachusetts founded in 1778. Both President George Herbert Walker Bush and his son President George Walker Bush graduated from Andover. So did 2018 Economics laureate Bill Nordhaus, a year behind me; he was a fellow member of the Radio Club, though I don’t remember him from then.
Education at Andover was rigorous in a patrician sort of way. Traditional skills like English composition were prominent in the curriculum, while I remember instruction in math and science to be rather old-fashioned. I was attracted to biology, whose head, Harper Follansbee, appointed in 1940, did nothing to discourage my herpetological ambitions. There was a magnificent indigo snake in one of the buildings. An ambitious young biology teacher, John Kimball, was appointed in 1956, and modernized the biology curriculum over the years. I barely remember him from my years at Andover, but have come to appreciate his great contribution to biology education, especially the free online textbook http://www.biology-pages.info/, which he continues to maintain to this day, and which I made liberal use of in my own teaching career.
Apart from Latin, French, and English, which I’ll discuss in the next section, my most memorable course at Andover was senior American history with Fred Allis. Memorable because Mr. Allis assumed a level of sophistication in the analysis of Supreme Court decisions, the economic theories of (I seem to remember) Joseph Schumpeter, etc., that was utterly beyond my abilities. Some of my classmates seemed to thrive on this stuff. I vaguely remember that a few even argued with Mr. Allis! Not me. Long, miserable evenings in the library earned me a (no doubt undeserved) gentleman’s C.
Altogether, though, I graduated from Andover well prepared for college – provided that I didn’t major in history.
Language
Three language classes at Andover were more memorable than American history: Latin, French, and senior English.
My Latin teacher was Frank Benton, who was appointed in 1918 and retired when I graduated in 1958. He looked upon our attempts to “construe” (translate out loud in class) our assigned Latin texts with curmudgeonly good humor. Wisely eschewing Cicero for the pimply teens in his third year Latin class, he assigned an up-to-date subject instead: medieval Latin. I hold our reader, Helen Waddell’s A Book of Medieval Latin for Schools, first published in 1931, before me as I write. The verse has understandable meter and clear rhymes that even a teen could appreciate. As I took up choral singing in college and afterward, thus regularly encountering medieval Latin, I came to appreciate Mr. Benton’s class even more.
My French teacher was James Grew, appointed in 1935. Mr. Grew had adopted la méthode directe. On the first day of French I, he briefly explained the rules of the class in English. That was the last English we were to hear from him for many months. He’d talk to us in French, ask us questions in French, sometime right in our faces, and eventually we’d start responding in French – broken French at first, of course, but gradually more and more idiomatic French. It was as if we were toddlers first learning our mother tongue – which is pretty much the logic of la méthode directe. French is the only foreign language in which I attained anything approximating fluency (mais maintenant j’ai presque tout oublié).
My senior English teacher was Dudley Fitts, appointed in 1941, who was in addition a prominent and well-respected poet, literary critic, and translator of classical Latin and Greek literature. What I remember most in his class was Chaucer, whose Middle English language we learned to understand and recite with some facility. I can’t say that senior English in general or Chaucer in particular had the same specific influence on my life as did French and Latin. But it did come in handy a few years ago, when our dinner group gathered for a meal whose theme was Spring. Someone had laboriously typed out the first 18 lines of Canterbury Tales, and asked if anyone would like to try reading it during the meal. “Sure,” I said, standing up and ignoring the typescript. I rendered those first 18 lines from memory, more than half a century after high school graduation, with (I flatter myself) dramatic flair and a creditable Middle English accent.
My engagement with language – both my own and foreign – has persisted to the present day. I continued to study French during my year in England as an exchange student between high school and college, and I took German and Spanish in college. I have learned to recognize roots in those and other languages, including some in Hebrew and Arabic. The language instinct has declined dramatically with age, of course; language is largely an academic enterprise now.
A year in England
Before going to college, I spent the 1958–1959 academic year as an exchange student at Wellington School, a combination day and boarding school in Wellington, Somerset, England. I was considered a Sixth Former (senior), and was assigned to one of the school’s houses (I don’t remember which one). By then I knew I was going to be a biology major in college, but I decided to take English, French, and History as my A-level subjects. Surprisingly considering what I wrote above about American history at Andover, I did OK in history: I think the History Master, whose name I believe was Victor Finn, was impressed by my writing ability, not my historical acumen. Evidently, I did pretty well in French too, because I have a copy of the Oxford Companion to English Literature with an inscription saying that it was the 1959 Modern Languages Prize.
An incident at Wellington School stands out as a shameful embarrassment in retrospect. Two representatives of South Africa’s apartheid government were invited to address a school assembly. For half an hour or so, one of them explained calmly and patiently how reasonable South Africa’s apartheid policy was, then asked for questions. I stood up and asked a respectful question – thank God I don’t remember what – in my Yank accent, which brought forth an amused comment from the speaker before he answered. After assembly, the History Master (again, I think Victor Finn) took some of us Sixth Formers aside and vented his fury that such an outrage had been allowed to occur in the school. I don’t remember him using the word “racism,” but I understand now that that was what he was denouncing. I felt mildly embarrassed at the time, but now I squirm at how unthinkingly I accepted such racist ideology as if legitimate.
Haverford College
My choice of Haverford College, a small Quaker school outside Philadelphia, counts as a sort of rebellion from my Andover background: Andover in those days was still largely a feeder school for Harvard, Yale, and Princeton.
I came just too late (September 1959) for any hope of a career in herpetology. Emmett “Dixie” Dunn, a prominent herpetologist, had been chair of the Biology Department, but he died in 1956. His replacement, Ariel Loewy, was even then (at age 34 in 1959) a prophet-like visionary on campus, whose soft-spoken charisma is still very much in evidence (along with a hacking cough) in an interview he gave in 1996 (Loewy, 1996). Loewy was born to a prosperous Jewish family in Bucharest, but the family fled to England in 1936 and then to Canada in 1941. He went to the University of Cambridge in England for a research fellowship in 1952–1953, and was thus eyewitness to Watson’s and Crick’s elucidation of the structure of DNA and the birth of the molecular genetics revolution. He accepted a position in Haverford’s Biology Department in 1953, and by the time I arrived had persuaded the college to let him utterly transform the curriculum, abandoning broad coverage of traditional subdisciplines in order to concentrate on cell and molecular biology. This was a unique educational choice at the time. It was also a terrific piece of luck for me: molecular biology was a far better fit to my abilities than herpetology, its math-like style congruent with the mathematical habit of mind I came to discover in myself at Haverford.
Loewy managed to recruit two ambitious young scientists who shared his curricular vision in full measure, and his charisma in some measure as well: Irv Finger and Mel Santer, who along with Loewy taught most of the biology courses I took.
In 2009 I reconnected with Santer, and told him about an incident in one of his courses that significantly influenced my scientific life. In one of his tests, he asked us (as I remember) to reconstruct the logic of an experiment by Howard Dintzis that had been published the year before (Dintzis, 1961), and that was immediately recognized as an important contribution to molecular biology. Dintzis showed that polypeptides are synthesized from amino terminus to carboxy terminus, by adding amino acids sequentially to the carboxy terminal end of the growing chain of amino acids. I was very pleased with my answer: I thought I’d caught on to the rationale of a complex experiment without a teacher’s help – an unusual or perhaps unique experience in my intellectual life to date. As I remember, Santer didn’t agree: he gave me a low mark on the question. Santer didn’t remember any of this in 2009, of course. Nevertheless, the “Dintzis experiment” (as it came to be called) reappeared in my life on several occasions, including in graduate school as I’ll explain below. This incident is emblematic of the many ways teachers can have lasting influences on their students without realizing it at the time.
A core component of Loewy’s curricular reform was the senior research tutorial, in which every senior biology major was required to complete a research project with one of the faculty. My senior year (1962– 1963) tutor was Meg Mathies, who served as temporary replacement for Santer during his sabbatical leave. Mathies was an immunologist who had just earned her Ph.D. under Abram Stavitsky at Case Western Reserve University; it was her influence that steered much of my subsequent research career toward molecular immunology.
My project was nothing if not ambitious: to show that the antigenbinding specificity of an antibody was determined by the messenger RNAs (mRNAs) that encode it. That seems like an obvious truth today, but it wasn’t then. The alternative template theory, which I’ll explain later in this essay, implied that the amino acid sequences of an antibody’s polypeptides (thus the nucleotide sequences of the corresponding mRNAs) did not suffice to explain its antigen specificity. My plan was to immunize a rabbit with a phage (phage T4, a virus that infects bacterial cells), extract RNA from the spleen (a primary immune organ) as the rabbit responded to the immunization, add that RNA to the cell-free protein synthesis system reported the year before (Nirenberg and Matthaei, 1961), and determine whether the resulting protein included antibodies that could neutralize T4 phages (that is, block their ability to infect bacterial cells). The experiment was hopelessly naïve. I failed to demonstrate any T4-neutralizing antibodies; it was Edgar Haber, who turned out to be my Ph.D. mentor a few years later, who defeated the template theory, as I’ll explain below. Still, a few things did work: the cell-free system did synthesize protein when artificial RNA was added in large amounts; T4 infection could be quantified using a plaque assay; the immunized rabbit did produce antibodies with strong T4-neutralizing activity as measured using the plaque assay. I used T4 to immunize rabbits and mice at several points in my later research career, and phage neutralization assays were key in my first phage-display publication (Smith, 1985).
I took a course called (I think) College Mathematics with a master teacher named Cletus Oakley. It explored a handful of carefully selected areas of mathematical analysis. I found this a most congenial kind of thinking, and went on to take the calculus sequence in college and a number of other courses afterward. Elementary math has been a significant component of my science throughout my career. Experimental results can often be presented in a more perspicuous, compelling, and workmanlike manner using simple mathematics. I can’t claim expertise in any area of the discipline, and I’m not an amateur do-it-yourself purist either. I cheerfully outsource challenging integrations or differential equations to online tables or utilities, or to colleagues who are actual mathematicians. My philosophical interest in probability theory, first encountered in Oakley’s class, as the fundamental rules of empirical reasoning (see below) arises from my engagement with math.
Haverford was still a Quaker school when I attended, and I admired the Quaker attitudes and commitment to social justice that I encountered in college. Many of my college friends had come to Haverford from Quaker schools. I participated in a number of Quaker weekend work camps in the inner city of Philadelphia. All this led to a short-lived engagement with Christianity (which had had little importance in my earlier life) during my college years, but religion dropped back out of my life for good after graduation. It has been Quakerism’s social attitudes and commitment to justice, not its devotional dimension, that has stuck with me.
Singing
During one of the summers in my college years, I got a tech job at the Marine Biological Laboratory in Woods Hole, on Cape Cod in Massachusetts. The college students like me spent many evening and weekend hours socializing, and I joined several other young people in an informal madrigal group. I have been singing in choruses ever since, with some interruptions. Years later, in 1978 in Columbia, Missouri, I was a founding member of the Ad Hoc Singers (a name we made up in haste before our first gig in a nursing home), which has evolved through several namechanges to the Columbia Chorale today. I’m still singing in the Chorale, progressing (regressing?) from second bass to second tenor as my low notes disappeared with age.
Engagement with choral singing meshes with my interest in language. I’ve written a number of musical essays for the Columbia Chorale, and I’ve posted an essay on Jerusalem of Gold on the Mondoweiss website. It is the language of the lyrics and how it relates to the musical diction that is my chief concern in these essays.
Briefly a high school teacher
I didn’t graduate from Haverford with an academic career in mind. Instead, my experience with the Quaker inner-city weekend work camps inspired me to be a high school teacher in neighborhoods like the ones I’d encountered. I took a few basic education courses in my senior year, and enrolled in the Master of Arts in Teaching (MAT) program at Temple University that summer. A semester of practice teaching in an inner-city school made me change my mind. I told myself that it was a teacher’s personal relations with young students, not engagement with their developing intellects, that was of prime importance, and I had little talent in that area. A more honest assessment might be my realization that teaching was going to be really hard work, and that maybe I should go for an easier life.
In any case, I resolved to apply to graduate school and took a temporary technician job with Martin Nemer at the Institute for Cancer Research (now the Fox Chase Cancer Center) in Philadelphia. Nothing could have locked in my new career choice more effectively than my brief experience there. Every afternoon there was a tea at which the whole institute, including many eminent scientists, were invited to socialize. As I remember, one of the regular participants, a man who made time for lowly techs like me, was Irwin Rose (he was awarded the Nobel Prize in Chemistry forty years later for the discovery of ubiquitin). This was heady stuff for a beginner!
Graduate school at Harvard Medical School and the Massachusetts General Hospital
In Fall 1964, I was admitted, along with about a dozen other students, to a PhD program at Harvard Medical School called (I think) the Biomedical Sciences (BMS) program. During our first year, we took an integrated basic medical sciences curriculum taught by a large team of professors, after which we would choose a dissertation mentor in any of a large selection of departments. BMS was a concentrated and remarkably comprehensive survey of modern biomedical research by master teachers. We students formed our own little society of friends, though in keeping with the army brat pattern of friendship I mentioned earlier I haven’t kept up with them.
I chose a cellular immunologist in the Department of Bacteriology and Immunology, Hugh McDevitt, as my dissertation advisor. I already had a project in mind: to use the “Dintzis experiment” that I had learned about in Santer’s class at Haverford (see above) to determine whether or not the variable (V) and constant (C) parts of an immunoglobulin polypeptide, which had been defined by the first immunoglobulin amino acid sequence results (Hilschmann and Craig, 1965), were synthesized as separate polypeptide chains that were subsequently joined together. (We now know, but didn’t then, that the V and C portions are joined in multiple steps at the chromosomal DNA level.) I didn’t get to embark on this project, however, since McDevitt was recruited to Stanford and I switched to the lab of Ed Haber (already mentioned above) at the Massachusetts General Hospital (MGH). “My” Dintzis experiment was published two years later by other immunologists, who showed that the V and C portions are synthesized as a single polypeptide (Knopf et al., 1967; Lennox et al., 1967). I wasn’t disappointed to be “scooped”; indeed, that established molecular immunologists would consider “my” experiment worth doing seemed to be a personal validation rather than a setback.
Haber, at age 34, was already chief of the Cardiac Unit at MGH (Editors, 1998) and an accomplished protein chemist. He had been born February 1, 1932, to a German Jewish family who escaped to Palestine in 1933 and moved to the U.S. in 1939. He had done his postdoc in the lab of Chris Anfinsen at the National Institutes of Health. Anfinsen was to receive the Nobel Prize in Chemistry in 1972 for showing that a protein’s primary structure (i.e., the amino acid sequence of its polypeptide) sufficed to specify its final three-dimensional (“folded”) structure, thus its biological activity. Haber had used Anfinsen’s methods to demonstrate the same principle in the case of antibodies (Haber, 1964). This put paid to the template theory (to be explained below) that had been the target of my failed senior tutorial project at Haverford.
Haber had a dreary basement lab in a dreary wing of the dreary (but storied) MGH. Sequencing immunoglobulins was a core experimental approach to molecular immunology at the time, and the Edman degradation was the flagship of sequencing technology. Machines called “sequenators” for automating the Edman degradation had been recently introduced. My plan was to develop a new kind of sequenator in which the protein to be sequenced would be exposed to the Edman reagents in the gas phase rather than dissolved in liquids. This innovation could have the important benefit of applying to small peptides that would be washed away by liquids. I had spectacular success at the beginning, but those successes could never be repeated, and the project was ultimately abandoned without any resulting publications.
The foregoing experimental failure wasn’t the end of my graduate career, for I had meantime been engaged in a theoretical study of the problem of “antibody diversity” (as we called it at the time). This problem arose in its starkest form from the work of Karl Landsteiner, who had shown in the early 20th century that animals were able to mount a specific antibody response to almost any synthetic organic chemical he tested (Landsteiner, 1962). “It is not reasonable that an animal produces predetermined antibodies against thousands of such synthetic substances,” Fritz Breinl and Felix Haurowitz had argued (Breinl and Haurowitz, 1930).
Breinl and Haurowitz’s solution to this conundrum, as elaborated later by Linus Pauling (Pauling, 1940), was the template theory. They proposed that a very limited number of generic antibody polypeptides could suffice for an unlimited number of different antibodies with distinct antigen-binding specificities. Antigen specificity arose (so went the theory) when these generic polypeptides first wrapped around the antigen as a template, then disengaged from the template as an antibody molecule with an antigen-specific binding site molded into its three-dimensional architecture. Antigen templates thus acted catalytically, each template molecule serving to stamp antigen-specificity into many antibody molecules. It’s the template theory that had been the target of my senior tutorial project at Haverford, and that Haber’s work had refuted (Haber, 1964). The great diversity of antibodies was now understood to reflect a corresponding great diversity of antibody-coding genes. And as the amino acid sequences of antibodies accumulated with increasing use of sequenators and other technical advances, it was clear that that diversity was concentrated in the V regions of their polypeptide chains.
My theoretical study focused on the evolution of V genes (the genes encoding V regions). Walter Fitch at the University of Wisconsin and I applied the computerized phylogenetic reconstruction algorithms he and Emanuel Margoliash had just developed (Fitch and Margoliash, 1967) to the increasing number of V region amino acid sequences that were becoming available. This is was the study at the core of my dissertation and a review by me, Leroy Hood, and Fitch (Smith et al., 1971). Two years later I extended these studies in a full-length monograph (Smith, 1973c). I used these analyses in a critical assessment of two competing explanations of V gene diversity: the somatic mutation and germline theories.
The somatic mutation theory supposed that the observed diversity of V region sequences arises through somatic mutation in V genes in individual B-cell clones during the lifetime of each individual animal or person (B cells are the lymphocytes that produce antibodies). According to this theory, the number of V region sequences far exceeds the number of germline V genes. The germline theory, in contrast, supposes that each possible V region an individual can express is directly encoded by a corresponding germline V gene. This theory required a large, though not infinite, number of V genes to account for the large number of different V region sequences. I argued that the evidence favored the germline theory, though not conclusively. Many predictions of, and arguments for, that theory have been confirmed by subsequent findings; in particular, there are indeed large numbers of V genes for some families of V regions. Nevertheless, the central claim of the germline theory has turned out to be completely wrong: extensive somatic mutation in individual B-cell clones is clearly the fundamental explanation for V region diversity.
My dissertation was finished in December 1969 with some help from my father, who used the drafting set he still had from West Point to draw several of the most complicated figures. The dissertation posed a problem for my committee: it reported no experimental results, my attempt at developing a new sequenator having failed. This was, to say the least, unusual, but I passed anyway, and got my degree at the beginning of 1970. Not for the last time in my career did I receive special treatment.
Haber wouldn’t put his name on any of these publications, but he was a very engaged mentor. In particular, he took care that I had a chance to interact with many other leaders in molecular immunology, an advantage that greatly enhanced my career.
War resistance
Between the Gulf of Tonkin resolution in August 1964 and the March on the Pentagon in October 1967, I became progressively more politically active against the war in Vietnam. Although I was too old for the military draft, I turned in my draft card to my local Selective Service board as a symbolic act of resistance. As was usual in those days, I was promptly drafted into the army, but refused induction very publicly in the presence of dozens of inductees in early 1968. This could well have resulted in a prison sentence, which would undoubtedly have greatly altered the course of my life. But as it turned out, I wasn’t imprisoned and suffered no other consequence of my act. The reason may be that the military was coming to realize that its policy of drafting resisters was backfiring. Refusing the draft was a highly visible form of resistance that was being effectively used to recruit young people to the anti-war cause.
Postdoc with Oliver Smithies at the University of Wisconsin
I first encountered Oliver Smithies at the 1967 Cold Spring Harbor Symposium on Antibodies, a landmark meeting at which major issues that were engaging immunologists at the time, including theories of antibody diversity, were vigorously discussed. Smithies presented a creative new theory of diversity, in which V-gene diversity was generated by extensive somatic recombination rather than somatic mutation. Francis Crick was effusive about Smithies’s hypothesis; he castigated the audience for not hailing the solution to the puzzle they had been seeking so long. A less modest person might have been tempted to gloat in triumph at praise from such high quarter. Not Smithies. He responded respectfully to the several participants who objected strenuously to his theory. I was more impressed with his integrity than Crick was with his theory.
I came to Smithies’s lab at the beginning of 1970. My main experimental project there (and completed at the University of Missouri) was sequencing the immunoglobulin light chains from a mouse myeloma tumor that was peculiar in secreting two light chains (Rose et al., 1977; Smith, 1973a, 1978b). One of them consisted of a signal sequence joined directly to the C region. We interpreted this as aberrant expression of an unjoined C gene, but the two light chains were later shown to be alternative splicing products of the primary RNA transcript of a single aberrantly joined light chain locus (Sikder et al., 1985).
Smithies was the one who in 1955 had introduced gel electrophoresis into the armamentarium of biological research in the form of starch gel electrophoresis, which was a regular component of our work in his lab. I’m one of the few living scientists who remembers the art of pouring, loading, running, and staining a starch gel. I say “art” advisedly, because success required mastery of skills that were exceedingly difficult to systematize in a manual: recognizing exactly the right “blurbling” sound as you boiled up the starch in a flask over an open flame, slapping a spatula just so onto the petroleum jelly covering the gel in order to peel it off in one piece after the run was over, etc. When Smithies turned his attention to DNA in collaboration with Fred Blattner toward the end of my postdoc, he devised an absolutely characteristic style of agarose gel electrophoresis. The molten gel was poured directly onto the benchtop, with a comb held in place by lumps of clay. After the DNA samples were loaded into the wells, warm petroleum jelly was poured over the surface to prevent evaporation (as in starch gel electrophoresis), and electrode trays were connected to the ends with paper wicks.
After the run, the petroleum jelly was removed with a spatula (again, as for starch gels) and the gel was lifted off the benchtop and stained with ethidium bromide – the final step being the only one that would be recognizable to today’s practitioners. Smithies was indeed an inveterate improviser. Otto Hiller, who made starch gel electrophoresis apparatuses in his shop in Madison, was a fellow tinkerer and Smithies’s regular Saturday afternoon companion, as he explains in his Nobel biography (Smithies, 2007). I admired but never emulated the improvisational style I witnessed in his lab.
The Genetics Building in Madison, where Smithies’s lab resided, was also home to two doyens of population genetics: Sewall Wright (December 21, 1889 to March 3, 1988), one of the founders of the field; and James Crow (January 18, 1916 to January 4, 2012), a master teacher and author of “Crow’s Notes,” a concise paperback guide for undergraduate genetics students, as well as coauthor with Motoo Kimura of the best-selling population genetics text An Introduction to Population Genetics Theory. Crow’s influence added a new dimension to my theoretical studies, which were continuing in Smithies’s lab. The germline theory of antibody diversity that I favored presumed that multiple germline V genes formed long tandem arrays in chromosomal DNA, as was already known for ribosomal RNA genes. Such arrays would be subject to occasional unequal crossover events in the germline, leading to repeated small contractions and expansions of the arrays over evolutionary time. Tandem genes subject to these expansions and contractions could be looked on as a population subject to many of the same principles of population genetics as a population of interbreeding organisms. This idea was included in my monograph on antibodies (Smith, 1973c) and presented at the 1973 Cold Spring Harbor Symposium (Smith, 1973b). My first publication from the University of Missouri was a lead article in Science arguing that any long stretch of chromosomal DNA not subject to natural selection would tend to turn into a tandem array, thus explaining the abundance of repetitive DNA with no obvious function in the genomes of many organisms (Smith, 1976).
University of Missouri and Columbia
In 1975 I was recruited to the newly-established Division of Biological Sciences at “Mizzou” by Abe Eisenstark, its first director. Eisenstark’s remit was a little like Loewy’s at Haverford: to modernize biological research and teaching on campus. I became part of a small coterie of young cell and molecular biologists who together constituted a favored establishment that was resented by the “Old Turks,” my name for a few older faculty who (with some justification) felt devalued in Eisenstark’s Risorgimento.
In my first few years at Mizzou I continued my experimental and theoretical study of antibody diversity, including the amino acid sequencing project I described above. My first doctoral student was Jamie Scott, who undertook to count the number of V genes in the mouse l family. A few dozen different V region sequences in this family had been published, but their diversity was severely limited. According to the somatic mutation theory, these V regions could have arisen from only two V genes; the germline theory, in contrast, would require dozens. Counting the mouse l V genes thus seemed a promising way to decide definitively between the somatic mutation and germline theories. Using two independent approaches, high-precision mathematical analysis of hybridization kinetics and denaturing gradient gel electrophoresis (Fischer and Lerman, 1980), Scott demonstrated that two genes accounted for all the V regions in the family, thus refuting the germline theory in this case (Scott et al., 1985). Her trip to Leonard Lerman’s lab in Albany to learn denaturing gradient gel electrophoresis, a lead pig with radioactive hybridization probe in her luggage, and the exciting results that ensued, are still fond memories. Scott’s article was never accepted for publication except as an abstract. One reason for this injustice is that the reviewers and editors believed, without justification, that the problem of antibody diversity had already been settled in favor of somatic mutation – an example of the impatient inattention to detail that is an unpleasant side-effect of the hurried pace of modern science. Scott returned to my lab as a postdoc after medical school, and played a key role in the development of that technology (Smith, 2018). She and her husband Felix Breden have been friends of my family ever since.
A few years after coming to Mizzou, I embarked on an ill-starred developmental biology project with the roundworm Caenorhabditis elegans, a model organism that my Biological Sciences colleague Don Riddle had brought from Sydney Brenner’s lab in Cambridge, England. My interest in filamentous phage biology arose from this project (Bauer and Smith, 1988; Crissman and Smith, 1984; Nelson et al., 1981; Smith, 1988; Zacher et al., 1980).
In August 1983, my wife Margie Sable started graduate school in the School of Public Health at the University of North Carolina. This was an opportunity for me to continue research in a prominent filamentous phage lab, Bob Webster’s at Duke University, only 10 miles away from Margie’s school. The first phage display experiment (Smith, 1985) was started in Bob’s lab at the end of my sabbatical year there.
The contribution of four coworkers to phage display was highlighted in my Nobel Prize lecture (Smith, 2018), and won’t be repeated here: Steve Parmley, Robert Davis, Jamie Scott (mentioned above), and Jinan Yu. But a number of other coworkers have undertaken phage-display projects that lay outside the scope of the lecture. Prominent among them were Valery Petrenko and Leslie Matthews.
Petrenko arrived as a visiting professor from Russia in 1993. The late professor Richard Perham of Cambridge University had run across his phage-display work in Novosibirsk (Minenkova et al., 1993a; Petrenko et al., 1991), and mediated an invitation to a Banbury Center conference in April 1992 (Minenkova et al., 1993b). Petrenko introduced an entirely new line of research in my lab: fashioning innovative “new materials” by engineering the major coat protein of filamentous virions (Petrenko and Smith, 2000; Petrenko et al., 1996; Petrenko et al., 2002); he has continued this research after moving to Auburn University as a professor in 2000. Petrenko and I have also collaborated on reviews and more conventional phage-display projects (Kouzmitcheva et al., 2001; Petrenko and Smith, 2005; Smith and Petrenko, 1997; Smith et al., 1998). Petrenko and his wife Natasha Petrenko have become friends of my family.
Leslie Matthews came to the lab as a doctoral student and stayed on as a postdoc. She and another postdoc, Melissa Nevils, were the lead scientists in the “epitope discovery” (ED) project that was my lab’s main research initiative for about five years starting in 1999. ED’s goal was to use phage display as a new gateway to discovery of promising synthetic vaccine candidates, especially for difficult diseases like malaria. After an auspicious start (Matthews et al., 2002), the project suffered a severe setback when Matthews’s and Nevils’s demonstration project with a malaria-like model, babesiosis of cattle, was not accepted for publication. Despite multiple attempts, I was unable to secure funding for applying the ED concept to human malaria.
My closest colleague in the Division of Biological Sciences has been Miriam Golomb. She is a gifted science writer who was one of the chief architects of the university’s Campus Writing Program – an endeavor to which she recruited Matthews, also a gifted writer. I myself taught many writing intensive courses. Golomb embraces an inspiring approach to undergraduate teaching that emphasizes strong student engagement, including regular extended conferences with students and lab courses with hands-on experiments that she’s constantly updating – recently adding a yeast CRISPR module, for instance.
For the final six years of my university career, my chief endeavor was a teaching initiative called Mathematics in Life Sciences (MLS), directed by math professor Dix Pettey. The program included a campus learning community called a freshman interest group for which I was faculty co-facilitator; and an alternative beginning biology lab that integrated elementary mathematics more intimately into the curriculum. Golomb and I developed the first MLS lab in Fall 2009, and I took charge of the Fall lab for the succeeding five years. The program was funded by the National Science Foundation for its first five years. Two of its lab modules have been published in a biology education journal (Smith, 2017; Smith et al., 2015).
I have had a long-standing philosophical and technical interest in probability theory as the fundamental guidebook for making rational scientific judgments about the world in light of the available evidence. This viewpoint goes by the name Bayesian statistics or philosophy because of the central role of Bayes’s theorem as the rule for updating our assessment of contending theories in the light of new evidence. Bayesian principles were included in a presentation I gave at a university genetics symposium in 1978 (Smith, 1978a), and in an article I co-authored with Hans Lehrach and his coworkers in Germany using Bitnet to exchange drafts (Michiels et al., 1987). I continue to talk and write informally about the subject.
My brother Mark and sister-in-law Lois Honeycutt also live in Columbia, where they’re history professors at the University of Missouri. My wife Margie was director of the School of Social Work until her retirement in 2016. Nepotism evidently isn’t a thing of the past at Mizzou.
Margie is Jewish, and since our marriage (next section) I’ve become increasingly engaged in Jewish culture. I’m not technically a Jew, however; that’s because for a non-believer like me, religious conversion would be dishonest. As I learned more and more about Zionism, I came to understand it as a great ongoing injustice against the Palestinian people, as well as a threat to Israeli Jews and to the wider Jewish society that I value as one of my most important adoptive communities. I helped organize Mid-Missourians for Justice in Palestine, a community organization that supports the global boycott, divestment, and sanctions (BDS) campaign against Israel until it ends its regime of subjugation and dispossession.
Tanksuit very much
I met Margie in October 1979 during a faculty/staff swim at the university’s natatorium. She was wearing a green tank suit, which lives on in our basement as an affectionate memento of our encounter. Soon after our romance began, she sent me a card with the title of this section as the printed message inside. We married October 10, 1981.

Figure 3. Margie and I welcome guests to our wedding party in American Legion Hall 202, October 10, 1981. It was a square dance, with no alcohol but plenty of pies. You can see the band in the background.
Our older son Alex Sable-Smith was born July 15, 1985 – the result of many plane trips between Columbia and Chapel Hill, where Margie had stayed on to continue her doctorate in public health after my sabbatical leave ended. I was the only solo male student in a child-birthing class in Columbia. Alex’s birth was a personal watershed, as fatherhood and family life in general grew to occupy at least as central a place in life as science. Alex is now a family physician, working in hospice and palliative medicine at the Veterans Administration Hospital in Palo Alto, California. Our younger son Bram Sable-Smith was born April 1, 1988. He’s now a freelance journalist in Madison, Wisconsin, where he lives with his wife Emma Brown.
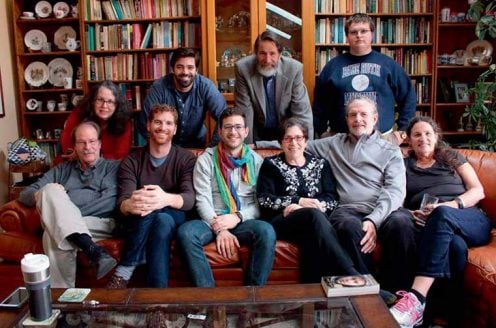
Figure 4. Family get-together, Christmas Day 2015. Seated: my brother Mark, Jesse Boyd (son of Matthew and Helen), our son Alex, Margie, my brother-law Matthew Boyd, and my sister Helen Boyd. Standing: Mark’s wife Lois Honeycutt, our son Bram, me, and Aubrey Smith (Mark and Lois’s son). Missing: Mark and Lois’s other son Derek, and Matthew and Helen’s daughter Casey.
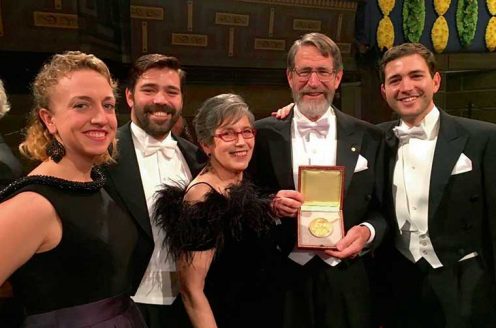
References
Bauer, M., and Smith, G.P. (1988). Filamentous phage morphogenetic signal sequence and orientation of DNA in the virion and gene-V protein complex. Virology 167, 166–175.
Breinl, F., and Haurowitz, F. (1930). Chemische Untersuchungen des Präzipitates aus Hämoglobin und Antihämoglobin-Serum und Bemerkungen über die Natur der Antikörper. Hoppe-Seylers Zeitschrift für physiologische Chemie 192, 45.
Crissman, J.W., and Smith, G.P. (1984). Gene-III protein of filamentous phages: evidence for a carboxyl-terminal domain with a role in morphogenesis. Virology 132, 445–455.
Dintzis, H.M. (1961). Assembly of the peptide chains of hemoglobin. Proceedings of the National Academy of Sciences of the United States of America 47, 247–261.
Editors (1998). In memoriam (for Edgar Haber). Bioconjug Chem 9, 3.
Fischer, S.G., and Lerman, L.S. (1980). Separation of random fragments of DNA according to properties of their sequences. Proceedings of the National Academy of Sciences of the United States of America 77, 4420–4424.
Fitch, W.M., and Margoliash, E. (1967). Construction of phylogenetic trees. Science 155, 279–284.
Haber, E. (1964). Recovery of Antigenic Specificity after Denaturation and Complete Reduction of Disulfides in a Papain Fragment of Antibody. Proceedings of the National Academy of Sciences of the United States of America 52, 1099–1106.
Hilschmann, N., and Craig, L.C. (1965). Amino acid sequence studies with BenceJones proteins. Proceedings of the National Academy of Sciences of the United States of America 53, 1403–1409.
Knopf, P.M., Parkhouse, R.M., and Lennox, E.S. (1967). Biosynthetic units of an immunoglobulin heavy chain. Proceedings of the National Academy of Sciences of the United States of America 58, 2288–2295.
Kouzmitcheva, G.A., Petrenko, V.A., and Smith, G.P. (2001). Identifying diagnostic peptides for lyme disease through epitope discovery. Clinical and Diagnostic Laboratory Immunology 8, 150–160.
Landsteiner, K. (1962). The Specificity of Serological Reactions, Revised Edition (New York, Dover Publications, Inc.).
Lennox, E.S., Knopf, P.M., Munro, A.J., and Parkhouse, R.M. (1967). A search for biosynthetic subunits of light and heavy chains of immunoglobulins. Cold Spring Harb Symp Quant Biol 32, 249–254.
Loewy, A.G. (1996). Michael Freeman interview with Ariel Loewy (http://hdl.handle.net/10066/1633).
Matthews, L.J., Davis, R., and Smith, G.P. (2002). Immunogenically fit subunit vaccine components via epitope discovery from natural peptide libraries. J Immunol 169, 837–846.
Michiels, F., Craig, A.G., Zehetner, G., Smith, G.P., and Lehrach, H. (1987). Molecular approaches to genome analysis: A strategy for the construction of ordered overlapping clone libraries. Comput Appl Biosci 3, 203–210.
Minenkova, O.O., Il’ichev, A.A., Kishchenko, G.P., Il’icheva, T.N., Khripin Iu, L., Oreshkova, S.F., and Petrenko, V.A. (1993a). [Preparation of a specific immunogen based on bacteriophage M13]. Molekuliarnaia Biologiia 27, 561–568.
Minenkova, O.O., Ilyichev, A.A., Kishchenko, G.P., and Petrenko, V.A. (1993b). Design of specific immunogens using filamentous phage as the carrier. Gene 128, 85–88.
Nelson, F.K., Friedman, S.M., and Smith, G.P. (1981). Filamentous phage DNA cloning vectors: a noninfective mutant with a nonpolar deletion in gene III. Virology 108, 338–350.
Nirenberg, M.W., and Matthaei, J.H. (1961). The dependence of cell-free protein synthesis in E. coli upon naturally occurring or synthetic polyribonucleotides. Proceedings of the National Academy of Sciences of the United States of America 47, 1588–1602.
Pauling, L. (1940). A theory of the structure and process of formation of antibodies. J American Chem Society 62, 2643–2657.
Petrenko, V.A., Kuzmicheva, G.A., Tatkov, S.I., Karpenko, L.I., and Ilyichev, A.A. (1991). Mutagenic properties of oligonucleotides with modified sugar-phosphate backbone. Nucleic Acids Symp Ser, 213–214.
Petrenko, V.A., and Smith, G.P. (2000). Phages from landscape libraries as substitute antibodies. Protein Engineering 13, 589–592.
Petrenko, V.A., and Smith, G.P. (2005). Vectors and modes of display. In Phage Display in Biotechnology and Drug Discovery, S.S. Siddhu, ed. (New York, CRC Press), pp. 63–110.
Petrenko, V.A., Smith, G.P., Gong, X., and Quinn, T. (1996). A library of organic landscapes on filamentous phage. Protein Engineering 9, 797–801.
Petrenko, V.A., Smith, G.P., Mazooji, M.M., and Quinn, T. (2002). Alpha-helically constrained phage display library. Protein Engineering 15, 943–950.
Rose, S.M., Kuehl, W.M., and Smith, G.P. (1977). Cloned MPC 11 myeloma cells express two kappa genes: A gene for a complete light chain and a gene for a constant region polypeptide. Cell 12, 453–462.
Scott, J.K., Smith, G.P., Lerman, L.S., and Gerlach, J. (1985). Cataloging germline immunoglobulin V-lambda genes by direct analysis of cellular DNA. Paper presented at: Advances in Gene Technology, Vol II: The Molecular Biology of the Immune System (Cambridge University Press).
Sikder, S.K., Kabat, E.A., and Morrison, S.L. (1985). Alternative splicing patterns in an aberrantly rearranged immunoglobulin kappa-light-chain gene. Proceedings of the National Academy of Sciences of the United States of America 82, 4045– 4049.
Smith, G.P. (1973a). Mouse immunoglobulin kappa chain MPC 11: Extra amino-terminal residues. Science 181, 941–943.
Smith, G.P. (1973b). Unequal crossover and the evolution of multigene families. Cold Spring Harb Symp Quant Biol 38, 507–513.
Smith, G.P. (1973c). The Variation and Adaptive Expression of Antibodies (Cambridge, MA, Harvard University Press).
Smith, G.P. (1976). Unequal crossover and the evolution of repeated DNA sequences. Science 191, 528–535.
Smith, G.P. (1978a). Non-Darwinian evolution and the beard of life. Stadler Symp 10, 105–110.
Smith, G.P. (1978b). Sequence of the full-length immunoglobulin kappa-chain of mouse myeloma MPC 11. The Biochemical journal 171, 337–347.
Smith, G.P. (1985). Filamentous fusion phage: Novel expression vectors that display cloned antigens on the virion surface. Science 228, 1315–1317.
Smith, G.P. (1988). Filamentous phage assembly: Morphogenetically defective mutants that do not kill the host. Virology 167, 156–165.
Smith, G.P. (2017). Understanding Reversible Molecular Binding. The American Biology Teacher 79, 746–752.
Smith, G.P. (2018). Nobel lecture in Chemistry, 2018: Phage display: Simple evolution in a Petri dish (https://www.nobelprize.org/prizes/chemistry/2018/smith/lecture/) (Stockholm, Sweden, Nobel Foundation).
Smith, G.P., Golomb, M., Billstein, S.K., and Montgomery Smith, S. (2015). The Luria-Delbrück Fluctuation Test as a Classroom Investigation in Darwinian Evolution. The American Biology Teacher 77, 614–619.
Smith, G.P., Hood, L., and Fitch, W.M. (1971). Antibody diversity. Annual Review of Biochemistry 40, 969–1021.
Smith, G.P., and Petrenko, V.A. (1997). Phage Display. Chemical Reviews 97, 391– 410.
Smith, G.P., Petrenko, V.A., and Matthews, L.J. (1998). Cross-linked filamentous phage as an affinity matrix. J Immunol Methods 215, 151–161.
Smithies, O. (2007). Biographical (https://www.nobelprize.org/prizes/medicine/2007/smithies/biographical/) (Stockholm, Sweden, Nobel Foundation).
Zacher, A.N.I., Stock, C.A., Golden, J.W.I., and Smith, G.P. (1980). A new filamentous phage cloning vector: fd-tet. Gene, 127–140.
This autobiography/biography was written at the time of the award and later published in the book series Les Prix Nobel/ Nobel Lectures/The Nobel Prizes. The information is sometimes updated with an addendum submitted by the Laureate.
Nobel Prizes and laureates
Six prizes were awarded for achievements that have conferred the greatest benefit to humankind. The 12 laureates' work and discoveries range from proteins' structures and machine learning to fighting for a world free of nuclear weapons.
See them all presented here.