Popular information
Information for the public (pdf)
Populärvetenskaplig information (pdf)
The Nobel Prize in Physiology or Medicine 2007
is awarded jointly to
Mario R. Capecchi, Martin J. Evans and Oliver Smithies
for their discoveries of “principles for introducing specific gene modifications in mice by the use of embryonic stem cells”
This year’s Nobel Laureates have discovered how to use embryonic stem cells to create precise and permanent genetic changes in mice. The mice generated in this manner are known as “knockout mice” because the change can lead to elimination (“knock-out”) of a gene function.
A two-part problem
Making specific genetic alterations that can be inherited is a two-part problem.
The first part is the challenge of finding a specific gene sequence among the three billion base pairs that comprise the human genome and then altering it in the intended way. Mario Capecchi and Oliver Smithies provided a solution to this problem when they demonstrated that homologous recombination can take place also in mammalian cells. Homologous recombination, which was already known to occur in other organisms, means that genes or gene segments within a cell can exchange information with each other.
That leaves the other part of the problem: ensuring that the specific alteration is passed down to all the cells in the next generation to make genetically modified mice. Here Martin Evans’ work with embryonic stem cells (ES cells) was of critical importance. He discovered and was able to culture these cells – cells that make up the early embryo and have a unique ability to develop into any cell type in the body. Evans discovered that if an alteration could be inserted into one of these cells, the same alteration would be found in all parts of the animal that developed from the embryonic stem cell.
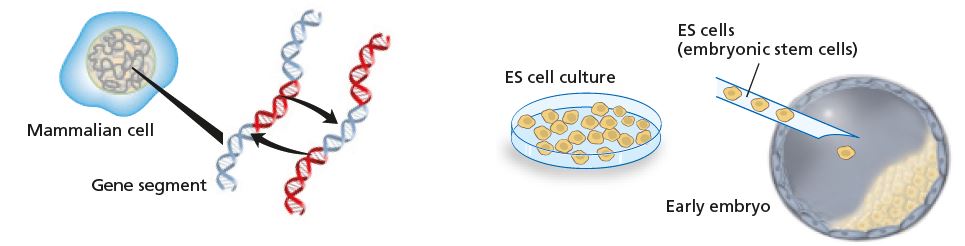
Capecchi and Smithies showed that homologous recombination can occur also in mammalian cells: genes or parts of genes can exchange places.
Evans showed that if a genetic modification is inserted into an ES cell that later gives rise to a new individual, the modification will be present in every cell of that new individual. © The Nobel Committee for Physiology or Medicine
Homologous recombination
All the information required for our bodies to develop and successfully interact with our surroundings is already stored in our genes when we are just a fertilized egg. The genetic material is packaged in the cell nucleus where the two-meter long DNA helix is organized into 23 pairs of chromosomes. One chromosome in every pair comes from each of our parents. When the cell divides, the chromosomes in the pair can exchange genetic information through homologous recombination.
Capecchi and Smithies had the vision that this type of genetic exchange could be used to introduce directed genetic changes in mammalian cells.

Creating targeted changes
In the early 1980s a research team led by Capecchi discovered that DNA sequences injected directly into the nucleus of a mammalian cell could be incorporated into the genes of the recipient cell – and through homologous recombination. The experiment inspired his idea that this technique could be used to make specific changes in any gene in a mammalian cell.
Eager to try out this new concept, he applied for funding from the National Institutes of Health. But the application was rejected: the grant reviewers considered it far too unlikely that the injected DNA would find its correct place in the gene pool of the target cell. (As it happens, Evans had a similar application rejected at about the same time. The UK Medical Research Council considered the idea overambitious!)
Smithies also wanted to make targeted changes, but his work focused mainly on attempts to repair defects in human blood cells. He discovered that the gene for the globin part of the blood protein hemoglobin could be altered using homologous recombination. Surprisingly, the change could be brought about regardless of whether the gene was switched on or not. This result suggested that it was possible to change any one of the genes in the gene pool at any time.

Lasting changes in the genome
The possibility of inserting new modifications into essentially any gene inspired new ideas. Capecchi realized that the technique could be used not only to repair defective DNA, but also to switch genes off.
However, the cell types in which Smithies and Capecchi had been able to make genetic changes did not give rise to germ cells. And without germ cells it was impossible to make genetic changes that could be inherited.
Meanwhile, however, Evans had published results showing inheritable genetic changes. A series of experiments done in his laboratory using embryonic tumor cells had not provided the results he sought. At that point he discovered that it was possible to grow cultures of cells with intact chromosomes from mouse embryos.
He found that these ES cells can differentiate into all the different types of cells in the body. That distinguishes ES cells from tissue stem cells. The latter lie dormant in various tissues and develop when there is a need for more cells of that type, but they cannot develop into any other cell type, the way ES cells can.

An early embryo is called a blastocyst. The cells in the outer layer (gray) will form the placenta. During a normal pregnancy, cells in the cell mass on the inside (green) would develop into an embryo.
These embryonic stem cells, ES cells, have not yet ifferentiated. This means they are still capable of developing into any of the cell types required to make up the new individual. © The Nobel Committee for Physiology or Medicine
Virus genes in mice
Provided it was possible to insert an altered gene into ES cells before they began to differentiate, the change ought to be present in every cell of the organism.
For this crucial experiment Evans used blastocysts, mouse embryos a few days old. He injected them with ES cells that had previously been infected with DNA from a virus. The embryos were implanted in the uterus of a surrogate mother, where they developed just like normal baby mice. When they were born it was possible to find viral DNA in the genome of some of the mouse pups. The experiments demonstrated that inserted DNA could in fact be passed down; this ground-breaking result was published in the journal Nature in October 1986.
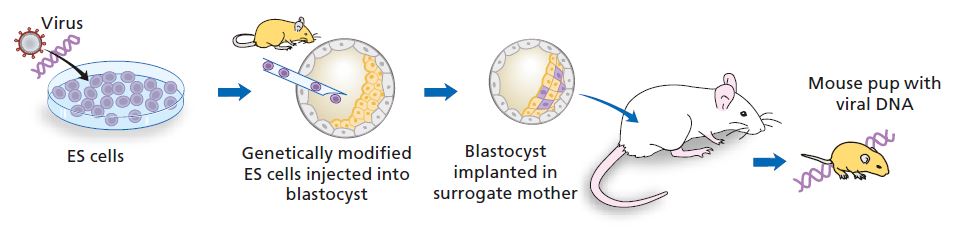
Putting the results together
In 1985 Capecchi had shown that homologous recombination actually did occur in mammalian cells, and Smithies has used this method to introduce altered DNA into a human cell. All this had been done in cells in culture.
When Evans published his results showing inheritance of viral DNA, all the pieces of the puzzle were on the table: it was time to try making targeted genetic changes in mice. The work proceeded on several fronts simultaneously. Evans was the expert on ES cells; Capecchi visited his laboratory over the Christmas holiday to learn how to handle these extraordinary cells, whereas Smithies received stem cells in a tube Evans had brought in his own pocket.
Both Smithies and Capecchi chose to create targeted changes in a gene that codes for an enzyme called hypoxanthine-guanine phosphoribosyltransferase (hprt). Mutations in the hprt gene result in a rare disease, Lesch-Nyhans syndrome, which is inherited through the X-chromosome. This means that women are carriers, but it is only men who have the disease.

An early embryo is called a blastocyst. The cells in the outer layer (gray) will form the placenta. During a normal pregnancy, cells in the cell mass on the inside (green) would develop into an embryo.
These embryonic stem cells, ES cells, have not yet ifferentiated. This means they are still capable of developing into any of the cell types required to make up the new individual. Photo: Jan Törnell. Illustration: © The Nobel Committee for Physiology or Medicine
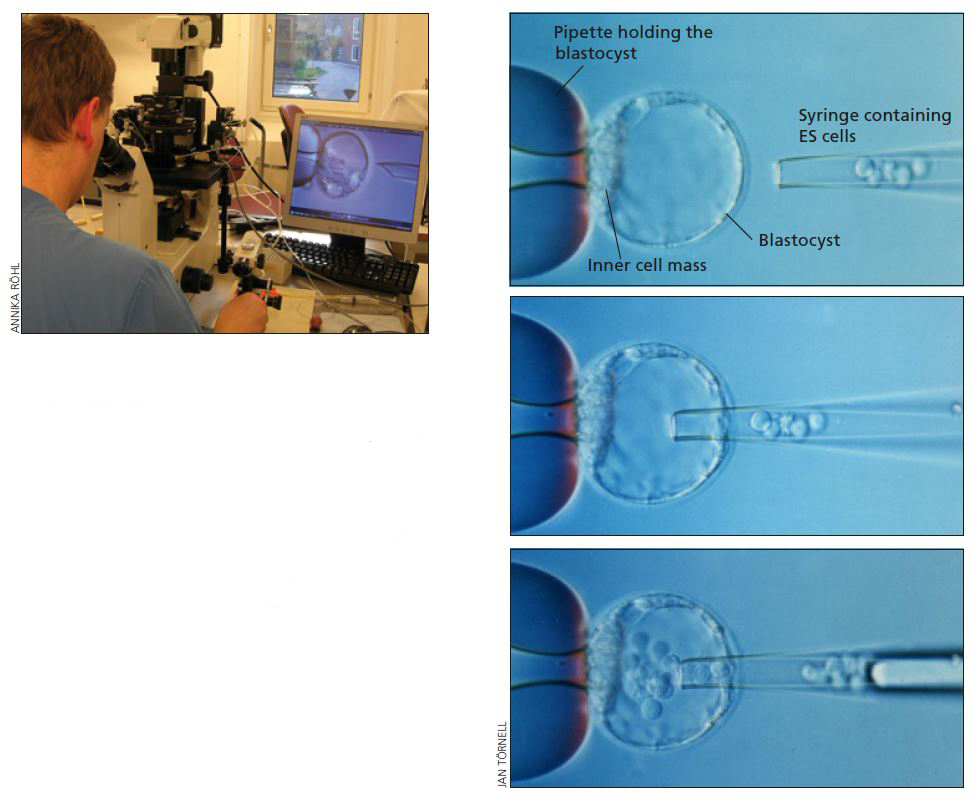
With the help of a phase contrast microscope, the researcher can do a microinjection, in-serting the modified ES cells into a blastocyst (above left).
The photographs on the right show how the blastocyst is held in place by a glass pipette. The modified ES cells are slowly injected through the glass syringe (right). Left photo: Annika Röhl. Right photos: Jan Törnell
Successful experiments
Smithies showed in 1987 that he could repair a defective hprt gene in cultured ES cells by injecting a new DNA fragment corresponding to an intact gene. The reason this particular gene was selected for the experiment was that a successful gene exchange was easy to detect: only the cells that had managed to take up the functional gene could grow in a special culture medium.
The same year Capecchi also succeeded in inserting a specific gene sequence into the hprt gene. This made the cells capable of surviving in a culture medium containing the antibiotic neomycin. In the journal Cell Capecchi mentions that this new technique would make it possible to create genetically modified mice. Many research groups took up this challenge and in 1989 several reports were published describing genetically modified mice. The new technique had come to stay.

New ways to select cells
A few years before this breakthrough, in 1986, Evans’ research group reported that they had managed to insert a special gene that enabled ES cells possessing the gene to survive and grow in culture medium containing neomycin. This was an important discovery, because it suggested a specific selection technique – positive selection – that could be used to pick out those few ES cells that had successfully taken up the new gene.
With Capecchi’s realization that negative selection was also required, the cell selection technique became truly elegant. Negative selection made it possible to get rid of cells bearing vectors that carried the resistance gene, but randomly inserted rather than after recombination with the target gene sequence.
Capecchi published his positive-negative selection method in 1988 in Nature. It was important, because homologous recombination occurs a thousand times less frequently than random insertion. A well-functioning selection method makes it possible to pick out the few cells in a cell culture that correctly carry the altered gene.
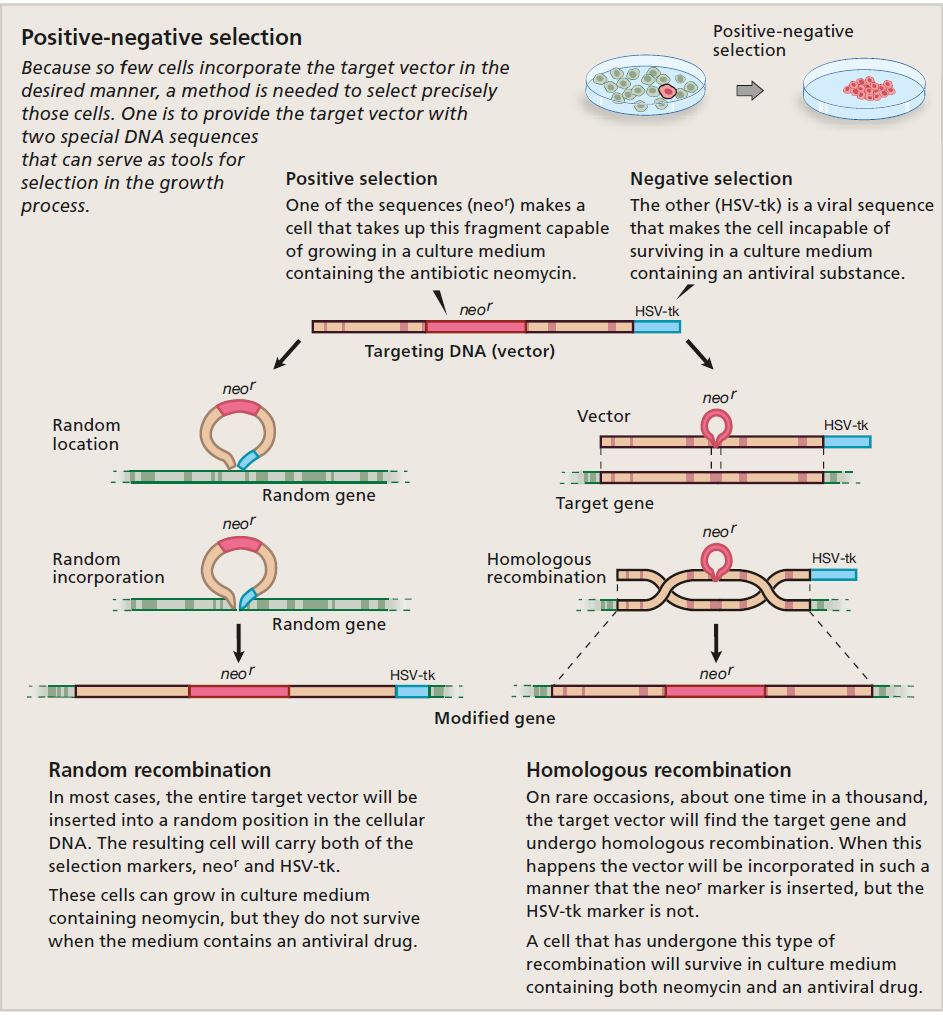
Over 10,000 genes have been modified
The technique of creating targeted changes in mice has been refined through the efforts of many scientists and is now widely used in biomedical research.
When the method was first presented many considered it so sophisticated and difficult to master that they believed it possible to use only for a handful of research questions. That was an overly pessimistic view. Today we have access to a multitude of different kinds of knockout mice; all in all, over 10,000 different genes have been modified. That corresponds to about half of all genes in humans and mice.
Modification does not necessarily mean that genes are knocked out; they can also be turned on (knock-on), have their function altered, or be replaced by another gene (knock-in).
The technique has now become so precise that it is possible make the inserted gene turn on or off only in a certain tissue, for instance blood cells or the nervous system, or in one of the many types of cells that make up the immune system.
It is now also possible to dictate at precisely what developmental stage a specific gene should become active or be turned off. The basis for this ingenious system is that the gene being introduced is given a special start sequence, in its so-called promoter region. This sequence requires addition of a specific substance before the gene can be read and initiate the formation of protein. The substance can be an antibiotic, for example, that the mouse receives at a specific time point.
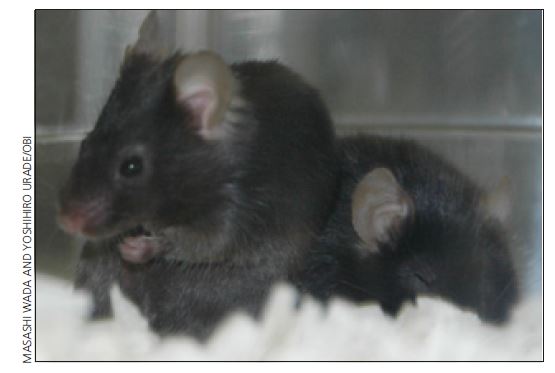
No ordinary transgenes
It should be emphasized that the technique for which this year’s Nobel Prize is awarded differs from that used to create transgenic mice, where DNA is injected into a fertilized egg. Such transgenic mice also have modifications in some of their genes. The difference is that with the older gene transfer technique, it is not possible to make targeted changes. This is because when altered DNA is injected into a fertilized egg it will usually be incorporated randomly into the genetic material. It is therefore impossible to control exactly where the change will end up, or how many copies of the new gene will be incorporated into the gene pool.
The transgene technique has been used to study what happens in the body when there are extra copies of a gene, something that leads to excess production of whatever protein that gene codes for. The fact that it is impossible to dictate the number of extra genes incorporated or where they are inserted limits the usefulness of this technique.
Realistic models
Using genetically modified mice, it has become possible to study how different genes function and interact, for example during embryonic development. But the technique is perhaps even more important in medical research because it now enables us to create models of a wide range of human diseases. Previously, genetic studies had to be carried out on cells in culture; but single cells never suffer from hypertension or diabetes. To gain insight into how common diseases like these develop and how they might be treated, more realistic models are needed. To date, there are over five hundred different types of genetically modified mice that mimic important human diseases such as atherosclerosis, hypertension and diabetes.

For example there are new mouse models for the study of cancer. It is now possible, in mice, to eliminate genes that usually counteract tumor development, such as the gene p53. In these animals, we can trace how cancer progresses and see which treatment is most effective against the cancer, and how this is affected by the stage at which the cancer is detected.
The complete genome was needed
This year’s prize is awarded to three scientists who did their pioneering work between 1981 and 1989. Why was there no prize for the knockout technique until this year? If the technology is to be used to confer benefit on mankind, it is crucial to be able to make changes in mouse genes with the same functions as those in humans. After all, we are two very different mammals. One important step forward in genetic research was taken in 2001, when the entire human gene pool – the genome – had at last been read. At the end of 2002 the first analysis of the mouse genome was completed. With this previously hidden information in hand, it was possible to make a complete comparison of the genes in mice and humans and to use this new knowledge in research.

Ethical considerations
This year’s prize also opens up for discussion of whether it is appropriate to use animals for research at all. Every experiment that involves animals is evaluated by an ethical review board appointed by the state; they take the potential suffering of the animals into consideration and weigh that against the potential future benefit. But the question remains: is it ethically defen- sible to create genetically modified mice using methods other than ordinary breeding? One strong argument is that by creating targeted changes in mice it is possible to establish better models of human disease, which can save human lives. In addition, better animal models mean fewer animals are needed to get research results, since the results are more reliable.
Modified mice that carry the human variant of a gene can also make drug tests more informative. One example is studies of receptors for the signaling substance serotonin, that is receptors involved in depressive disorders. The human forms of these receptors differ from those in both mice and rats. Previously, researchers did not have any good animal models. Now it is possible to study how a new drug works in mice who carry human serotonin receptors.
The Laureates
Mario R. Capecchi was born in Italy in 1937 but is now a US citizen. In 1967 he received his PhD in Biophysics at Harvard University, Cambridge, Massachusetts. He is a Howard Hughes Medical Institute Investigator and Professor of Human Genetics and Biology at the University of Utah, Salt Lake City, Utah, USA.
Since 1989 one of Capecchi’s research interests has been to study which genes are active during the embryonic development and he has been able to show which genes guide anatomic for- mation and how different organs develop.
Sir Martin J. Evans was born 1941 in Great Britain and is a British citizen. He received his PhD in Anatomy and Embryology in 1969 at University College, London. He is now Director of the School of Biosciences and Professor of Mammalian Genetics at Cardiff University.
Evans has created models of human diseases, including several mouse models of cystic fibrosis.
Oliver Smithies was born in 1925 in Great Britain but is now a US citizen. In 1951 he received a PhD in Biochemistry at Oxford University, UK. He is currently Professor of Pathology and Laboratory Medicine at the University of North Carolina at Chapel Hill, USA.
Smithies, like Evans, has used this technique to create disease models of cystic fibrosis and the hematological disease thalassemia, as well as models for hypertension and atherosclerosis.
Previous Nobel Prizes in this research field
This year’s award touches on aspects of several previous Nobel Prizes that have gone to research on how the genome is organized, how genes exchange information with each other, and the molecular biological techniques that make it possible to produce specific DNA sequences.
1933: Thomas Morgan “for his discoveries concerning the role played by the chromosome in heredity”.
1958: Joshua Lederberg “for his discoveries concerning genetic recombination and the organization of the genetic material of bacteria”.
1962: Francis Crick, James Watson and Maurice Wilkins “for their discoveries concerning the molecular structure of nucleic acids and its significance for information transfer in living material”.
1968: Robert Holley, Har Gobind Khorana and Marshall Nirenberg “for their interpretation of the genetic code and its function in protein synthesis”.
1978: Werner Arber, Daniel Nathans and Hamilton Smith “for the discovery of restriction enzymes and their application to problems of molecular genetics”.
2006: Andrew Fire and Craig Mello “for their discovery of RNA interference – gene silencing by double-stranded RNA”.
In addition to these Nobel Prizes in Physiology or Medicine, research in this field has been awarded Nobel Prizes in Chemistry.
1980: Paul Berg “for his fundamental studies of the biochemistry of nucleic acids, with particular regard to recombinant-DNA” and Walter Gilbert and Frederick Sanger “for their contributions concerning the determination of base sequences in nucleic acids”.
1993: Kary Mullis and Michael Smith “for contributions to the developments of methods within DNA-based chemistry”, to Kary Mullis “for his invention of the polymerase chain reaction (PCR) method”, and to Michael Smith “for his fundamental contributions to the establishment of oligonucleotide-based, site-directed mutagenesis and its development for protein studies”.
The editorial committee for this year’s popular presentation of the Nobel Prize in Physiology or Medicine included the following, who are professors at Karolinska Institutet and members of the Nobel Assembly:
Christer Betsholtz, Bertil Fredholm, Göran K. Hansson, Hans Jörnvall and Nils-Göran Larsson
Text: Lotta Fredholm, science journalist and medical editor
Translation: Janet Holmén, editor
Illustrations: Bengt Gullbing and Annika Röhl
© 2007 The Nobel Committee for Physiology or Medicine 2007, Karolinska Institutet, 171 77 Stockholm
Nobel Prizes and laureates
Six prizes were awarded for achievements that have conferred the greatest benefit to humankind. The 12 laureates' work and discoveries range from proteins' structures and machine learning to fighting for a world free of nuclear weapons.
See them all presented here.