Advanced information
Scientific Background:
The Brain’s Navigational Place and Grid Cell System (pdf)
Scientific Background
The Brain’s Navigational Place and Grid Cell System
The 2014 Nobel Prize in Physiology or Medicine is awarded to Dr. John M. O’Keefe, Dr. May-Britt Moser and Dr. Edvard I. Moser for their discoveries of nerve cells in the brain that enable a sense of place and navigation. These discoveries are ground breaking and provide insights into how mental functions are represented in the brain and how the brain can compute complex cognitive functions and behaviour. An internal map of the environment and a sense of place are needed for recognizing and remembering our environment and for navigation. This navigational ability, which requires integration of multi-modal sensory information, movement execution and memory capacities, is one of the most complex of brain functions. The work of the 2014 Laureates has radically altered our understanding of these functions. John O’Keefe discovered place cells in the hippocampus that signal position and provide the brain with spatial memory capacity. May-Britt Moser and Edvard I. Moser discovered in the medial entorhinal cortex, a region of the brain next to hippocampus, grid cells that provide the brain with an internal coordinate system essential for navigation. Together, the hippocampal place cells and the entorhinal grid cells form interconnected nerve cell networks that are critical for the computation of spatial maps and navigational tasks. The work by John O’Keefe, May-Britt Moser and Edvard Moser has dramatically changed our understanding of how fundamental cognitive functions are performed by neural circuits in the brain and shed new light onto how spatial memory might be created.
Introduction
The sense of place and the ability to navigate are some of the most fundamental brain functions. The sense of place gives a perception of the position of the body in the environment and in relation to surrounding objects. During navigation, it is interlinked with a sense of distance and direction that is based on the integration of motion and knowledge of previous positions. We depend on these spatial functions for recognizing and remembering the environment to find our way.
Questions about these fundamental brain functions have engaged philosophers and scientists for a long time. During the 18th century the German philosopher Immanuel Kant (1724-1804) argued that some mental abilities exist independent of experience. He considered perception of place as one of these innate abilities through which the external world had to be organized and perceived.
A concept of a map-like representation of place in the brain was advocated for by the American experimental psychologist Edward Tolman, who studied how animals learn to navigate (Tolman, 1948). He proposed that animals could experience relationships between places and events and that the exploration of the environment gradually resulted in the formation of a cognitive map that enabled animals to navigate and find the optimal path through the environment. In this view, cognitive maps represent the environment as a gestalt that allows the subject to experience the room and navigate.
Tolman’s theory opposed the prevailing view among behaviourists that complex behaviours are achieved by chains of sensory-motor response relationships. But it did not address where in the brain these functions may be localized and how the brain computes such complex behaviours. The advent of techniques to record from cells in the brain of animals that were freely moving in the environment, using chronically implanted micro wires (Sturmwasser, 1958), made it possible to approach these questions.
Finding the place cells
John O’Keefe had a background in physiological psychology, working with Ronald Melzack at McGill University before he moved to the laboratory of the pain researcher Patrick Wall at University College in London, where he started his work on behaving animals in the late 1960s. There he discovered the place cells, when recording from neurons in the dorsal partition of hippocampus, called CA1, together with Dostrovsky, in rats moving freely in a bounded area (O’Keefe and Dostrovsky, 1971) (Figure 1).
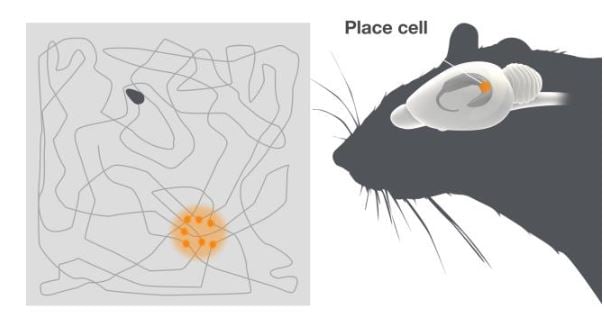
Figure 1. Place cells. To the right is a schematic of the rat. The hippocampus, where the place cells are located is highlighted. The grey square depicts the open field the rat is moving over. Place cells fire when the animal reaches a particular location in the environment. The dots indicate the rat’s location in the arena when the place cell is active. Different place cells in the hippocampus fire at different places in the arena.
The firing pattern of these cells was completely unexpected. Place cells were active in a way that had not been seen for any cells in the brain before. Individual place cells were only active when the animal was in a particular place in the environment, namely their place field. By systematically changing the environment and testing different theoretical possibilities for the creation of the place fields O’Keefe showed that place cell firing did not merely reflect activity in sensory neurons, but that it represented a complex gestalt of the environment.
Different place cells could be active in different places and the combination of activity in many place cells created an internal neural map representing a particular environment (O’Keefe, 1976; O’Keefe and Conway, 1978). O’Keefe concluded together with Nadel that place cells provide the brain with a spatial reference map system, or a sense of place (O’Keefe and Nadel, 1978). He showed that the hippocampus can contain multiple maps represented by combinations of activity in different place cells that were active at different times in different environments. A specific serial combination of active place cells may therefore represent a unique environment, while other combinations represent other environments. Through O’Keefe’s discoveries, the cognitive map theory had found its representation in the brain.
A prerequisite for O’Keefe’s experiments was the development of appropriate recording techniques to be used in freely moving animals. Although O’Keefe was an early user of these techniques, he was not the first to record from hippocampal or other nerve cells in intact animals (see O’Keefe and Nadel 1978). However, researchers mostly used restricted behavioural task or strict stimulus-response protocols. In contrast, O’Keefe recorded the cellular activity during natural behaviour, which allowed him to observe the unique place fields and relate the neural activity in the place cells to represent the sense of place.
In subsequent experiments, O’Keefe showed that the place cells might have memory functions (O’Keefe and Conway, 1978; O’Keefe and Speakman, 1987). The simultaneous rearrangement in many place cells in different environments was called remapping and O’Keefe showed that remapping is learned, and once it is established, it can be stable over time (Lever et al., 2002). The place cells may therefore provide a cellular substrate for memory processes, where a memory of an environment can be stored as specific combinations of place cells.
At first, the proposition that the hippocampus was involved in spatial navigation was met with some scepticism. However, it was later appreciated that the discovery of place cells, the meticulous demonstration that these cells represent a mental map far from primary sensory input, and the proposal that hippocampus contains an inner map that can store information about the environment, were seminal. O’Keefe’s discovery sparked a large number of experimental and theoretical studies on how place cells are engaged in generating spatial information and in spatial memory processes. The general notion from these studies is that the key function of the place cells are to create a map of the environment, although they may also be involved in measuring distance under some circumstances (Ravassard et al., 2013).
From hippocampus to grid cells in the entorhinal cortex
Through the 1980s and 1990s the prevailing theory was that the formation of place fields originated within the hippocampus itself.
May-Britt Moser and Edvard Moser, who were studying the hippocampus, both during their PhD work in Per Andersen’s laboratory in Oslo and afterwards both as visiting scientists in Richard Morris’ laboratory in Edinburgh and John O’Keefe’s laboratory in London, asked whether the place cell firing can be generated from activity outside hippocampus. The major input to the hippocampus comes from a structure on the dorsal edge of the rat’s brain, the entorhinal cortex. A large part of the output from the entorhinal cortex projects to the dentate gurus in hippocampus, which in turn connect to the region in the hippocampus called CA3, and further to CA1 in the dorsal hippocampus. Interestingly, this is the same the part of the brain in which John O’Keefe first found the place cells. In 2002, the Mosers found that disconnecting projections from the entorhinal cortex through CA3 did not abolish the CA1 place fields (Brun et al., 2002). These findings, and the knowledge that medial entorhinal cortex is also directly and reciprocally connected to the CA1 region, prompted May-Britt Moser and Edvard Moser to look in the medial entorhinal cortex for place coding cells. In a first study they established, similar to what others had shown, that the medial entorhinal cortex contained cells that shared characteristics with the place cells in hippocampus (Fyhn et al., 2004). However, in a later study using larger encounters for the animals to move in, they discovered a novel cell type, the grid cells, that had unusual properties, (Hafting et al., 2005).
The grid cells showed an astonishing firing pattern. They were active in multiple places in the open box that together formed nodes of an extended hexagonal grid (Figure 2), similar to the hexagonal arrangements of holes in a beehive.

Figure 2. Grid cells. The grid cells are located in the entorhinal cortex depicted in blue. A single grid cell fires when the animal reaches particular locations in the arena. These locations are arranged in a hexagonal pattern.
Grid cells in the same area of the medial enthorinal cortex fire with the same spacing and orientation of the grid, but different phasing, so that together they cover every point in the environment.
The Mosers found that the distance of the grid fields varies in the medial entorhinal cortex with the largest fields in the ventral part of the cortex. They also showed that the grid formation did not arise out of a simple transformation of sensory or motor signals, but out of complex network activity.
The grid pattern had not been seen in any brain cells before! The Mosers concluded that the grid cells were part of a navigation or path integration system. The grid system provided a solution to measuring movement distances and added a metric to the spatial maps in hippocampus.
The Mosers further showed that grid cells were embedded in a network in the medial entorhinal cortex of head direction cells and border cells, and in many cases, cells with a combined function (Solstad et al., 2008). Head-direction cells were first described by James Ranck (1985) in another part of the brain, the subiculum. They act like a compass and are active when the head of an animal points in a certain direction. Border cells are active in reference to walls that the animal encounters when moving in a closed environment (Solstad et al., 2008; Savelli, et al. 2008). The existence of border cells was predicted by theoretical modelling by O’Keefe and colleagues (Hartley, et al. 2000). The Mosers showed that the grid cells, the head direction cells, and the border cells, projected to hippocampal place cells (Zhang et al. 2013). Using recordings from multiple grid cells in different parts of the entorhinal cortex, the Mosers also showed that the grid cells are organized in functional modules with different grid spacing ranging in distance from a few centimetres to meters, thereby covering small to large environments.
The Mosers further explored the relationship between grid cells and place cells in theoretical models Solstad et al., 2006), lesion experiments (Bonnevie et al., 2013; Hafting et al., 2008), and in remapping experiments (Fyhn et al. 2007). These and other studies by Mosers and O’Keefe, as well as by others, have shown that there is a reciprocal influence between grid cells in the medial entorhinal cortex and place cells in the hippocampus and that other spatially- tuned cells in the entorhinal cortex, in particular the border cells (Figure 3), may contribute in the generation of the firing pattern of the place cells (Brandon et al., 2011; Koenig et al., 2011; Bush, Berry and Burgess, 2014, Bjerkness et al. 2014).
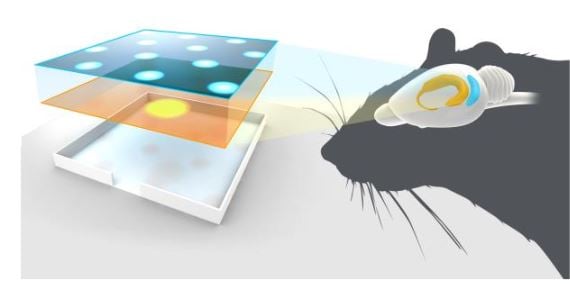
Figure 3. A schematic showing grid cells (blue) and place cells (yellow) in the entorhinal cortex and hippocampus, respectively.
The Mosers’ discovery of the grid cells, a spatial metric coordination system, and their identification of the medial entorhinal cortex as a computational centre for spatial representation, is a break-through that opens up new avenues to advance the understanding of the neural mechanisms underlying spatial cognitive functions.
The grid and place cell systems are found in many mammalian species including humans
Since the initial description of place and grid cells in rat and mice, these cell types have also been found in other mammals (Killian et al., 2012; Ulanovsky et al., 2007; Yartsev et al., 2011, 2013;). Humans have large hippocampal-entorhinal brain structures and these structures have long been implicated in spatial learning and episodic memory (Squire, 2004). A number of studies support the idea that the human brain has a spatial- coding system that is similar to that found in non-human mammals. Thus, researchers have found place-like cells in the hippocampus (Ekstrom et al., 2003; Jacobs et al., 2010) and grid-like cells in the entorhinal cortex (Jacobs et al., 2013) when directly recording from nerve cells in the human brain of patients with epilepsy undergoing pre-surgical investigation. Using functional imaging (fMRI). Doeller et al. (2010) have also provided support for the existence of grid cells in the human entorhinal cortex.
The similarity of the hippocampal-entorhinal structure in all mammals and the presence of hippocampal-like structures in non- mammalian vertebrates with navigational capacity suggest that the grid-place cells system is a functional and robust system that may be conserved in vertebrate evolution.
The importance of the discovery of place cells and grid cells for research in cognitive neuroscience
It is an emergent theme that place-coding cells in the hippocampal structures are involved in storing and/or retrieving spatial memories. In the 1950s Scoville and Milner (1957) published their report on the patient Henry Molaison (HM), who had his two hippocampi surgically removed for treatment of epilepsy. The loss of hippocampi caused severe memory deficits, as evident by the clinical observation that HM was unable to encode new memories, while he could still retrieve old memories. HM had lost what has later been named episodic memory (Tulving and Markowitch 1998), referring to our ability to remember self-experienced events. There is no direct evidence that place cells are coding episodic memory. However, place cells can encode not only for the current spatial location, but also where the animal has just been and where it is going next (Ferbinteanu and Shapiro, 2003). The past and present may also be overlapping in time in place cells when animals are rapidly tele-transported between two physical different environments (Jezek et al., 2011). An encoding of places in the past and present might allow the brain to remember temporally ordered representations of events, like in the episodic memory.
After a memory has been encoded, the memory undergoes further consolidation, e.g. during sleep. Ensemble recording with multi-electrodes in sleeping animals has made possible the study of how memories of spatial routes achieved during active navigation are consolidated. Groups of place cells that are activated in a particular sequence during the behaviour display the same sequence of activation in episodes during the subsequent sleep (Wilson and McNaughton, 1994). This replay of place cell activity during sleep may be a memory consolidation mechanism, where the memory is eventually stored in cortical structures.
Together the activity of place cells may be used both to define the position in the environment at any given time, and also to remember past experiences of the environment. Maybe related to this notion is the findings that the hippocampus of London taxi drivers, which undergoes extensive training to learn how to navigate between thousands of places in the city without a map, grew during the year long training period and that the taxi drivers after this training had significantly larger hippocampal volume than control subjects (Magurie et al. 2000, Woollett and Maguire, 2011).
Relevance for humans and medicine
Brain disorders are the most common cause of disability and despite the major impact on people’s life and on the society, there is no effective way to prevent or cure most of these disorders. The episodic memory is affected in several brain disorders, including dementia and Alzheimer’s disease. A better understanding of neural mechanisms underlying spatial memory is therefore important, and the discoveries of place and grid cells have been a major leap forward to advance this endeavour. O’Keefe and co-workers have showed in a mouse model of Alzheimer’s disease that the degradation of place fields correlated with the deterioration of the animals’ spatial memory (Cacucci et al., 2008). There is no immediate translation of such results to clinical research or practice. However, the hippocampal formation is one of the first structures to be affected in Alzheimer’s disease and knowledge about the brain’s navigational system might help understand the cognitive decline seen in patients with this diseases.
Conclusions
The discoveries of place and grid cells by John O’Keefe, May-Britt Moser and Edvard I. Moser present a paradigm shift in our understanding of how ensembles of specialized cells work together to execute higher cognitive functions. The discoveries have profoundly promoted new research with grid and place cell systems now found in many mammals, including humans. Studies of the navigation system have opened new avenues for studying how cognitive processes are computed in the brain.
Ole Kiehn and Hans Forssberg Karolinska Institutet
Ole Kiehn, MD, PhD
Professor of Neuroscience, Karolinska Institutet
Member of the Nobel Committee
Member of the Nobel Assembly
Hans Forssberg, MD, PhD
Professor of Neuroscience , Karolinska Institutet Adjunct
Member of the Nobel Committee
Member of the Nobel Assembly
Illustrations: Mattias Karlén
Cited literature
Bjerknes, T.L., Moser, E.I. and Moser, M.B. (2014). Representation of geometric borders in the developing rat. Neuron, 82(1), 71-78.
Bonnevie, T., Dunn, B., Fyhn, M., Hafting, T., Derdikman, D., Kubie, J.L., Roudi, Y., Moser, E.I., and Moser, M.B. (2013). Grid cells require excitatory drive from the hippocampus. Nature Neuroscience 16, 309-317.
Brandon, M.P., Bogaard, A.R., Libby, C.P., Connerney, M.A., Gupta, K., and Hasselmo, M.E. (2011). Reduction of theta rhythm dissociates grid cell spatial periodicity from directional tuning. Science 332, 595-599.
Brun, V.H., Otnass, M.K., Molden, S., Steffenach, H.A., Witter, M.P., Moser, M.B., and Moser,
E.I. (2002). Place cells and place recognition maintained by direct entorhinal-hippocampal circuitry. Science 296, 2243-2246.
Bush. D., Barry, C., Burgess, N. (2014). What do grid cells contribute to place cell firing? Trends in Neuroscience, 37(3), 136-145
Cacucci, F., Yi, M., Wills, T.J., Chapman, P. and O´Keefe, J. (2008) Place cell firing correlates with memory deficits and amyloid plaque burden in Tg2576 Alzheimer mouse model. PNAS, 105, 7863-7868.
De Hoz, L., and Wood, E.R. (2006). Dissociating the past from the present in the activity of place cells. Hippocampus, 16, 704-715.
Doeller, C.F., Barry, C., and Burgess, N. (2010). Evidence for grid cells in a human memory network. Nature 463, 657-661.
Ekstrom, A.D., Kahana, M.J., Caplan, J.B., Fields, T.A., Isham, E.A., Newman, E.L., and Fried, I. (2003). Cellular networks underlying human spatial navigation. Nature 425, 184-188.
Ferbinteanu, J., and Shapiro, M.L. (2003). Prospective and retrospective memory coding in the hippocampus. Neuron, 40, 1227-1239.
Fyhn, M., Hafting, T., Treves, A., Moser, M.B., and Moser, E.I. (2007). Hippocampal remapping and grid realignment in entorhinal cortex. Nature 446, 190-194.
Fyhn, M., Molden, S., Witter, M.P., Moser, E.I., and Moser, M.B. (2004). Spatial representation in the entorhinal cortex. Science 305, 1258-1264.
Hafting, T., Fyhn, M., Bonnevie, T., Moser, M.B., and Moser, E.I. (2008). Hippocampus- independent phase precession in entorhinal grid cells. Nature 453, 1248-1252.
Hafting, T., Fyhn, M., Molden, S., Moser, M.B., and Moser, E.I. (2005). Microstructure of a spatial map in the entorhinal cortex. Nature 436, 801-806.
Hartley, T., Burgess, N., Lever, C., Cacucci, F. and O’Keefe, J. (2000). Modeling place fields in terms of the cortical inputs to the hippocampus. Hippocampus, 10(4), 369-379.
Jacobs, J., Kahana, M.J., Ekstrom, A.D., Mollison, M.V., and Fried, I. (2010). A sense of direction in human entorhinal cortex. PNAS 107, 6487-6492.
Jacobs, J., Weidemann, C.T., Miller, J.F., Solway, A., Burke, J.F., Wei, X.X., Suthana, N., Sperling, M.R., Sharan, A.D., Fried, I., and Kahana, M.J. (2013). Direct recordings of grid-like neuronal activity in human spatial navigation. Nature Neuroscience, 6, 1188-1190.
Jezek, K., Henriksen, E.J., Treves, A., Moser, E.I., and Moser, M.B. (2011). Theta-paced flickering between place-cell maps in the hippocampus. Nature, 478, 246-249.
Killian, N.J., Jutras, M.J., and Buffalo, E.A. (2012). A map of visual space in the primate entorhinal cortex. Nature 491, 761-764.
Langston, R.F., Ainge, J.A., Couey, J.J., Canto, C.B., Bjerknes, T.L., Witter, M.P., Moser, E.I., and Moser, M.B. (2010). Development of the spatial representation system in the rat. Science 328, 1576-1580.
Lever, C., Wills, T., Cacucci, F., Burgess, N., and O’Keefe, J. (2002). Long-term plasticity in hippocampal place-cell representation of environmental geometry. Nature 416, 90-94.
Maguire, E.A., Gadian, D.G., Johnsrude, I.S., Good, C.D., Ashburner, J., Frackowiak, R.S. and Frith C.D. (2000). Navigation-related structural change in the hippocampi of taxi drivers. PNAS, 97(8), 4398-4403.
O’Keefe, J. (1976). Place units in the hippocampus of the freely moving rat. Experimental neurology 51, 78-109.
O’Keefe, J., and Conway, D.H. (1978). Hippocampal place units in the freely moving rat: why they fire where they fire. Experimental brain research 31, 573-590.
O’Keefe, J., and Dostrovsky, J. (1971). The hippocampus as a spatial map. Preliminary evidence from unit activity in the freely-moving rat. Brain research 34, 171-175.
O’Keefe, J., and Nadel, L. (1978). The Hippocampus as a Cognitive Map (Oxford University Press ).
O’Keefe, J., and Speakman, A. (1987). Single unit activity in the rat hippocampus during a spatial memory task. Experimental brainresearch 68, 1-27.
Ranck, J.B. (1985). Head direction cells in the deep cell layer of dorsal presubiculum in freely moving rats. In Electrical Activity of the Archicortex, C.V. G. Buzsáki, ed. (Budapest: Akademiai Kiado), pp. 217-220.
Ravassard, P., Kees. A., Willers, B., Ho, D., Aharoni, D., Cushman, J., Aghajan, Z.M. and Mehta
M.R. (2013) Multisensory control of hippocampal spatiotemporal selectivity. Science,
340(6138), 1342-1346.
Sargolini, F., Fyhn, M., Hafting, T., McNaughton, B.L., Witter, M.P., Moser, M.B., and Moser, E.I. (2006). Conjunctive representation of position, direction, and velocity in entorhinal cortex. Science 312, 758-762.
Savelli, F., Yoganarasimha, D and Knierim, J.J (2008). Influence of boundary removal on the spatial representations of the medial enthorinal cortex. Hippocampus, 18, 1270-1282.
Scoville, W.B., and Miller, B. (1957). Loss of recent memory after bilateral hippocampal lesions.
Journal of Neurology Neurosurgery and Psychiatry, 20, 11-21.
Solstad, T., Boccara, C.N., Kropff, E., Moser, M.B., and Moser, E.I. (2008). Representation of geometric borders in the entorhinal cortex. Science 322, 1865-1868.
Solstad, T., Moser, E.I., and Einevoll, G.T. (2006). From grid cells to place cells: a mathematical model. Hippocampus 16, 1026-1031.
Squire, L.R. (2004). Memory systems of the brain: a brief history and current perspective.
Neurobiology of learning and memory 82, 171-177.
Stensola, H., Stensola, T., Solstad, T., Froland, K., Moser, M.B., and Moser, E.I. (2012). The entorhinal grid map is discretized. Nature 492, 72-78.
Strumwasser, F. (1958). Long-term recording from single neurons in brain of unrestrained mammals. Science, 127, 469-670.
Tolman, E.C. (1948). Cognitive maps in rats and men. Psychological Review, 55, 189-208.
Tulving, E. and Markowitsch, H.J. (1998). Episodic and declarative memory: role of the hippocampus. Hippocampus, 8, 198-204.
Ulanovsky, N., and Moss, C.F. (2007). Hippocampal cellular and network activity in freely moving echolocating bats. Nat Neurosci 10, 224-233.
Wilson, M.A., and McNaughton, B.L. (1994). Reactivation of hippocampal ensemble memories during sleep. Science 265, 676-679.
Woollett K. and Maguire E.A. (2011). Acquiring “the Knowledge” of London’s layout drives structural brain changes. Current. Biology, 21(24), 2109-2114
Yartsev, M.M., and Ulanovsky, N. (2013). Representation of three-dimensional space in the hippocampus of flying bats. Science 340, 367-372.
Yartsev, M.M., Witter, M.P., and Ulanovsky, N. (2011). Grid cells without theta oscillations in the entorhinal cortex of bats. Nature 479, 103-107.
Zhang, S.J., Ye, J., Miao, C., Tsao, A., Cerniauskas, I., Ledergerber, D., Moser, M.B., and Moser,
E.I. (2013). Optogenetic dissection of entorhinal-hippocampal functional connectivity. Science 340, (6128)232627.
Nobel Prizes and laureates
See them all presented here.