Tasuku Honjo
Biographical
Prologue
Burning wooden walls collapsed in front of us. I was on the back of my mother, who was running away from our burning house, gasping for air. Although I was just three and a half years old, I vividly remember this scene that seems to be engraved forever in my memory. It was one of the most devastating American bombing raids targeting civilians, which took place in Toyama City on August 1, 1945, just two weeks before the end of the war. We escaped the fire by jumping into a ditch beside a rice field to avoid the heat from the nearby burning houses. Just minutes before, an incendiary bomb had hit the bomb shelter in our garden where we had been hiding. Luckily, that bomb did not explode. I believe I was extremely fortunate from this early stage of my life.
I was born in Kyoto on January 27, 1942, less than two months after World War II started in the Pacific region. My family traces its ancestry back to a priest from a temple called Honjo-san Sensho-ji. Many years ago, when visiting the temple for the first time, I immediately recognized my relatives among others without any introduction. Their familiar eyes, eyebrows, noses and cheeks resembled those of my immediate family members.
We were welcomed by the head priest, Kuniyuki Honjo, who showed us the Sensho-ji Temple and writings about its origins. We were told that the Temple had been established sometime between 1077 to 1080 by Goromaru Fujiwara, a guard who helped the third son of the Emperor Go-Sanjo escape fighting in Kyoto, then Japan’s capital.
My father worked as a surgeon at the Kyoto University Hospital. He later became the head of the Otorhinolaryngology Department in the Yamaguchi University Medical School in Ube City, where he served until his retirement in 1976. Having spent several years in Montreal’s McGill University and Northwestern University in Chicago learning advanced surgical operations, he was fluent in English and perceived it was the new lingua franca one needed to master. Thus, my father introduced me to Ms. Kato, a Japanese woman born in Hawaii who taught me English two years ahead of my classmates, which helped my communication skills later in my career. My father enjoyed painting and was also an excellent golf player. I seem to have inherited his athletic but not artistic traits, although I do appreciate and enjoy paintings and music. While my father educated me rather strictly, my mother was protective and warm, forever my guardian goddess. She not only saved my life but shaped the person I was to become with her love (Fig. 1).
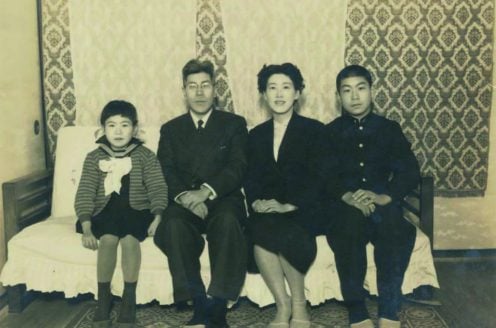
Figure 1. Honjo family in 1955. From left to right Nobuko, Sho-ichi, Ryuko and Tasuku.
My first moment of enchantment with the natural sciences took place when I observed the tiny rings around Saturn in a clear sky using a portable telescope in the playground of Kamihara Elementary School in Ube during a summer vacation. Afterwards, I began to read many astronomy books and was determined to be an astronomer. My passion for astronomy continued for a few years until my mother gave me the biography of Hideyo Noguchi, a world-famous yet unconventional Japanese microbiologist. Noguchi obtained his medical doctor’s license while struggling with incredible poverty and also a physical handicap after seriously burning his hand. He went to Philadelphia at the age of 24 to meet the great pioneer in the infectious disease research Simon Flexner, who later hired Noguchi at the Rockefeller Medical Institute. Noguchi is mostly known for his finding that progressive paralytic disease is caused by infection of the brain with syphilis, and for his pursuit of the cause of yellow fever. Ironically, the latter was to cause his sudden death in Ghana.
When I first had to make decisions about my future path at the end of senior high school, I was still uncertain. I knew I did not want to be a clerk or a civil servant, because I wanted to do something I was passionate about. I considered becoming a lawyer or embracing a diplomatic career, because I thought I could communicate effectively. However, the medical profession was very attractive to me, as not only my father but many of our relatives were clinical doctors. In the end, I chose to continue this legacy and applied for entry to the Kyoto University Medical School.
During the years around 1960, as I entered medical school, I felt I was witnessing a revolution in biology, with discoveries such as the double helix structure of DNA in 1953 and the decoding of the triplet nature of the genetic code in 1964 by Marshall Nirenberg and Philip Leder. I recall being extremely impressed by a Japanese book called Revolution in Biology by Atsuhiro Shibatani, who happened to be a colleague of my father at Yamaguchi University. Published in 1960, the book contained amazing speculations: that cancer is a manifestation of mutations in our DNA; that automated DNA sequencing will enable us to define these mutations; that the development of ‘molecular surgery’ will allow us to repair defects in DNA and may ultimately cure cancer. The night after I was exposed to Shibatani’s extraordinary imagination, I could not sleep. I was totally immersed and fascinated by biochemistry and molecular biology, which I believed would lead to a better understanding of what life is.
Early training
Fortunately for my scientific career, I became bored with memorizing Latin names for anatomy and the symptoms of many diseases with unknown causes in medical school and began to spend most of my time in the laboratory of Professor Osamu Hayaishi. Hayaishi was a great biochemist – the discoverer of oxygenase enzymes – who had just returned to Kyoto University from a nine-year stint at the National Institutes of Health (NIH) and Washington University in St. Louis. He worked with the biochemist and Nobel Laureate Arthur Kornberg, with whom he kept a close friendship throughout his life. I eagerly joined Hayaishi’s department for graduate studies between 1967–1971. During this time, and also later in my life, I learned much from Hayaishi – not only about science but also other enjoyable pastimes like golf and wine tasting, which later gave me much pleasure. In Hayaishi’s lab, I was mentored by Yasutomi Nishizuka, who was then famous for elucidating how the molecule NAD, essential for energy production in all living cells, is synthesized from the amino acid tryptophan, and later discovered protein kinase C and its regulation by phorbol ester.
I believe it was in the summer of 1967 when I found a very interesting paper by John Collier demonstrating the requirement for NAD by diphtheria toxin for inactivation of protein synthesis enzyme EF-2. This work was striking, as at that time toxins were thought to function by directly binding components of cellular machinery to block their activity. I had a strong desire to find out why NAD is essential for diphtheria toxin’s function. I was, fortunately in the best place to answer this question, due to the availability of labeled forms of NAD generated by Nishizuka’s research on the NAD pathway. I quickly established that diphtheria toxin is an enzyme that catalyzes transfer of the ADP-ribose moiety of NAD to EF-2 and published this finding in the prestigious Journal of Biological Chemistry (Honjo et al., 1968). Publishing this work while still a graduate student gave me a boost of confidence. The remaining two years of my graduate course were, sadly, scientifically lost, as student riots forced the closure of the university campus. I was already thinking of the next step. Following the example of my mentors and my father before me, I was determined to challenge myself in an international scientific environment.
I was fortunate to be hired as a postdoctoral fellow at the Carnegie Institution of Washington in Baltimore. The beautiful Carnegie building was located next to the northwest corner of the Homewood campus of Johns Hopkins University. Retrospectively, an incident which changed my life occurred about one year after my arrival. Donald Brown gave a seminar in Carnegie about the organization of the immunoglobulin (Ig) gene. At that time, not only immunologists but all biologists were keen to find how animals generate specific antibodies against almost every foreign antigen they encounter. Two major hypotheses were put forward to explain the enormous diversity of antibodies produced by B lymphocytes. The first so-called ‘germline’ hypothesis claimed that we may have as many as one thousand genes responsible for the antibody diversity phenomenon. The second theory, called ‘somatic hypermutation’ originally proposed by Sir Frank Macfarlane Burnet, claimed that DNA mutations in immune cells could generate enormous lymphocyte diversification from a limited number of inherited genes. A major problem with the germline hypothesis was explaining how individual copies of the Ig variable (V) gene could differ so greatly while maintaining the important structural information contained in the Ig constant (C) region gene. Based on his studies on the organization of ribosomal RNA genes, Don proposed that multiple copies of the C gene might be conserved by frequent homologous recombination between other copies of C genes to repair errors and suggested that this long-standing mystery of biology could be approached with modern molecular biological technology. I could not stop asking Don where the best place to tackle this fundamental problem was. He suggested Philip Leder, whom I met three months later when he gave a seminar in Carnegie. My long journey in molecular immunology began when Phil accepted me to his lab at NIH.
The work in Phil’s lab was exciting every day. There was a movable arrow on the wall pointing either to the germline or somatic hypermutation theory. The strategy Phil proposed was to count the number of the light (L)-chain C genes by hybridization kinetics, using radiolabeled cDNA derived from purified Ig L chain messenger RNA. The speed with which these labeled genetic probes were reannealed after denaturation, together with total cellular DNA, would reflect the number of L-chain C genes present in the genome. Our conclusion was that there is only one copy or very few copies of the Ig Lk or Ll chain gene. Ironically, these results ran contrary to Don’s preferred germline theory of diversity.
Back to Japan
With this result, after almost four years in America I felt drawn back to Japan. Phil advised me to stay, as in 1974, it was apparent that the Japanese universities had neither money nor the sophisticated facilities to carry out advanced molecular biological studies. I faced the second big choice in my life. I finally made up my mind to go back to Japan. Firstly, I wanted my small children (my daughter, Yasuko who had recently been born in Baltimore, and my five-year-old son, Hajime) to experience aspects of Japanese culture that might be lost to them if we continued to live abroad, and secondly, I wanted to nurture a strong culture of molecular biology research into Japan, as my mentor Hayaishi had done for biochemistry. I am happy to have seen my children thrive back in Japan, Hajime as a gastroenterologist and Yasuko as an embryologist, although for their success I owe a debt of gratitude to my wife Shigeko as I was a typical, workaholic Japanese husband. Phil accepted my decision and kindly recommended me for a small grant from the Jane Coffin Childs Memorial Fund, which was instrumental in launching my career at the University of Tokyo. He also generously sent precious RNA reverse transcriptase, an essential reagent to generate cDNA that was then difficult to obtain.
The University of Tokyo was considered the best university in Japan. However, I found that not only the infrastructure but also the spirit of science was far behind what I had experienced in Hayaishi’s laboratory. To avoid direct competition with Phil, I decided to reorient my work on the Ig heavy (H)-chain gene structures that had their own unique biological question, i.e., how different classes of antibody like IgG, IgE or IgA were made during immune responses. Although the question was clear, I faced many technical hurdles as even a basic piece of equipment like a gel dryer was difficult to find. I remember going down to the Akihabara area – where many shops sell electronic components – to buy thick rubber plates, hose and mesh to build my own vacuum drying apparatus for electrophoresis gels, which were essential for visualizing proteins. A few brilliant medical students – who later took the graduate course in my lab – voluntarily worked after classes with me.
It took almost three years until we began to obtain interesting data. Surprisingly, we found that the gamma chain constant (Cγ) gene was deleted from DNA in myelomas. To examine whether CH gene deletion was an artifact of the myeloma cancer we were studying, I asked Michael Potter – an expert in plasma cell cancers working at NIH – to send more myeloma cells producing different Ig classes. Tohru Kataoka and I carried out extensive Cot Curve analyses of many myeloma DNAs (Fig. 2). At that time, I was commuting from Yokohama to Tokyo, which took 90 minutes each way. One night, while poring over results on the train on my way back home, I found that the CH gene deletion always takes place upstream the CH gene expressed in that particular myeloma, assuming a specific order of CH genes on the chromosome. That was the moment when I reached the DNA deletion hypothesis model for class switch recombination (CSR) (Honjo and Kataoka, 1978). I was extremely delighted when our research was highlighted by the Nature editor Miranda Robertson, in that journal’s News and Views.
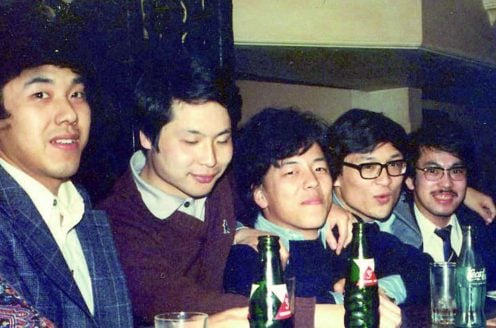
Figure 2. Students in Tokyo around 1979. From left to right, Tohru Kataoka, Yoshio Yao-ita, Naoki Takahashi, Toshiaki Kawakami and Akira Shimizu.
In 1977, I returned to Phil’s lab for three months when they restarted experiments after the lifting of a long, frustrating moratorium on gene cloning technology. I quickly succeeded in isolating Cγ1 cDNA, starting from purified mRNA using molecular cloning techniques, with the help of John Seitman. I was fascinated when we established the sequence of the Cγ1 gene, and finally discovered that each domain of the Cγ1 chain is separated by noncoding spacers. Akira Shimizu and others went on to map all the CH genes on the mouse chromosome (Fig. 2). We were very much delighted to prove that the proposed DNA deletion model of antibody diversity was absolutely correct. The next clear goal was to elucidate the mechanism in detail, and especially to identify the enzyme(s) that catalyze(s) CSR.
Journey to aid
In 1979, five years after I came back from Phil’s laboratory, I was invited to become the head of the Department of Genetics at Osaka University School of Medicine. Elevating a relatively early-stage researcher to such a prominent position was something unprecedented in Japan. However, my stay in Osaka was short, as in 1984 I was invited back to Kyoto University to succeed Hayaishi after his retirement. A highlight during my time in Osaka was being invited to the Nobel symposium organized by Erna Möller and Göran Möller in 1982. There I met Eva Severinson, who later proposed a collaboration to clone cytokines which could enhance class switching, as she had established a mouse helper T cell line and a sensitive experimental assay system for class switching. By expressing a fulllength cDNA library in amphibian oocytes, we discovered IL-4 and IL-5, which turned out to be critical for not only CSR but also T cell differentiation.
In 1991, I was invited to NIH as a Fogarty Scholar, a very valuable sabbatical opportunity. It provided me with a year’s salary and residence within the NIH campus, to encourage interactions with its many scientists. I enjoyed staying at the NIH campus and talking with many immunologists, including William Paul, who provided an education in cellular immunology, especially regulation of the immune response. One day I met Warren Strober and his associate Yoshio Wakatsuki from Kyoto University, who told me they had a cell line that could be stimulated to switch from producing IgM to IgA antibodies in vitro. Instantly, I realized this cell line could be what I was looking for in order to identify the enzyme(s) responsible for induction of CSR. After repeatedly selecting efficiently switching clones for two years, we finally isolated a cell line (CH12F3) that robustly expressed IgA after 48-hour stimulation with CD40 ligand, IL-4 and TGF-β1.
Masamichi Muramatsu began looking for differences in cDNAs expressed by CH12F3 cells before and after stimulation (Fig. 3). One gene that caught his attention was expressed only in activated B cells but not T cells. In addition, the gene was turned on in germinal centers, the active sites in the lymphoid tissues where CSR was known to take place. Surprisingly, the clone closely resembled genes in the cytidine deaminase family, including RNA editing enzyme APOBEC-1. Indeed, Muramatsu could show it had noticeable if weak cytidine deaminase activity on free cytidine. We published a paper on the isolation of the activation induced cytidine deaminase (AID) gene in 1999 in the Journal of Biological Chemistry. Privately, I had chosen to name the gene AID because both aid and Tasuku can mean “help”, which I did not tell Masamichi. We continued to investigate the AID function by knocking out the gene in mice. By the end of January 2000, we could show that AID-deficient animals lacked both CSR and SHM (Muramatsu et al, 2000).
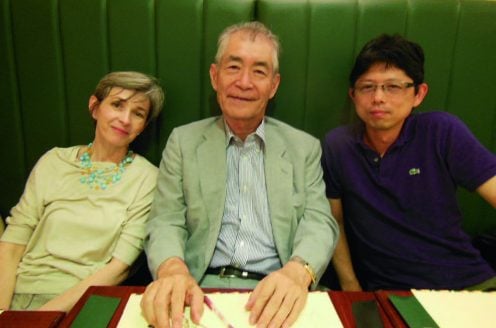
Figure 3. From left to right, Sidonia Fagarasan, Tasuku Honjo and Masamichi Muramatsu in 2013.
We had been asked for collaboration by Anne Durandy and Alain Fischer from Hôpital Necker-Enfants Malades in Paris, who were trying to identify the gene responsible for a severe immune deficiency in patients with hyper-IgM syndrome type 2 (HIGM II). These patients had very high concentrations of IgM in their blood, strongly suggesting a genetic defect in class switching. We sent primers to Paris to detect the AID gene, which their group used to identify AID mutations in all HIGM II patients. They also found HIGM II patients were defective in SHM. We quickly confirmed that this process was also lacking in AID-deficient animals. The result was completely unexpected, as the scientific consensus at the time was that SHM and CSR were completely different modifications carried out by different genes. However, these findings showed that the AID protein alone was responsible for these two critical genetic alterations in Ab genes during immune responses. It was a eureka moment for me and the entire group working on AID. At this time, serving as a dean of the Medical School of Kyoto University, I was afflicted with very severe back pain, to the point of not being able to walk or sit comfortably. I worked on the AID paper lying on the floor in my office while colleagues kneeled next to me to discuss plans and ongoing experiments. I remember the year 2000 for both the physical challenge and some of the most exciting scientific breakthroughs in my life.
I presented this result at the Annual Meeting of the American Association of Immunologists in Seattle in May 2000, after we sent the two manuscripts to Cell describing AID deficiency in mice and humans. The lecture was well accepted by a large audience. Although it was clear that the game was over in the hunt for the enzymes responsible for CSR and SHM, for us this was the beginning of another journey to find out how such a small protein can mediate two complicated genetic changes in immune cells.
Discovery of PD-1, its function and application
The mechanisms of clonal selection, namely, how immature T cells are selected during development in the thymus, was another big question in immunology. In my lab, Yasumasa Ishida was striving to identify the molecule(s) responsible for the thymic T cells selection, and proposed comparing the gene expression of growing and dying cells from the thymus (Fig. 4). In May 1991, he used this technique to identify a gene expressed during this process which we named PD(programmed cell death)-1 (Ishida et al., 1992). The structure predicted from the gene sequence indicated that PD-1 is a surface receptor protein, related to but distinct from known receptor molecules involved in lymphocyte activation. Therefore, I thought we should focus on finding the function of this protein in immune cells. Hiroyuki Nishimura generated knock-out (KO) mice on a mixed genetic background in June 1994, but to our disappointment the KO mice were identical to controls (Fig. 4). Fortunately, Nagahiro Minato, a very knowledgeable and experienced tumor immunologist in our faculty, suggested that we should breed the PD-KO mice to create an inbred strain and continue to monitor the mice before drawing any conclusion (Fig. 5). Thanks to Minato’s guidance, by November 1997, we observed that when introduced to the autoimmune-prone lpr/lpr strain, PD-1 deficiency led to arthritis and nephritis at five months of age. Continuing our patient monitoring, we found that PD-1 deficiency on the C57BL/6N background caused mice to develop similar autoimmune symptoms at 14 months of age. These findings convinced us that PD-1 is a negative regulator of the immune system (Nishimura et al, 1999). Disease manifestation in PD-1 KO mice was milder than that of CTLA-4 KO mice, all of which were reported to die within 4–5 weeks. The CTLA-4 gene, originally discovered by Pierre Golstein, was shown to encode a negative immune-receptor by Jeff Bluestone, Craig Thomson, Jim Allison, Tak Mak and Arlene Sharpe. The comparatively mild and chronic symptoms in PD-1 KO mice suggested to us that PD-1 could safely be targeted to treat diseases. Around March 1998, we began a series of experiments targeting PD-1 as a therapy for various disorders characterized by defects in immune regulations, such as cancer, infection, autoimmunity, and rejection of transplanted organs. Reasoning that blocking PD-1 would be simpler than enhancing its function, boosting the immune response against cancer became our first goal. Yoshiko Iwai began work looking ways to use PD-1 in therapeutic applications and generated many reagents and experimental systems (Fig. 4).
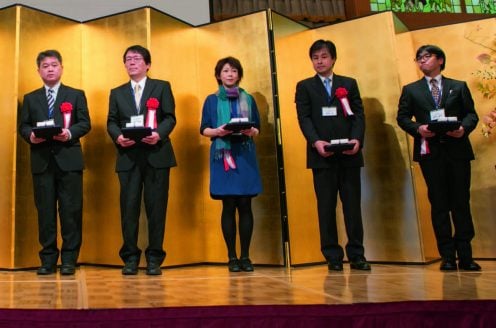
Figure 4. From left to right, Yasutoshi Agata, Yasumasa Ishida, Yoshiko Iwai, Taku Okazaki, Hiroyuki Nishimura at the celebration party of the CHAOO award by the Japanese Can-cer Association in 2014.
Ever since discovering PD-1, we had predicted the presence of a specific binding partner or ligand (Ishida et al., 1992). On September 17, 1998, we spoke with Steve Clark, the head of the Genetic Institute, about our problem with identification of ligands for PD-1. He proposed using their Biacore instrument to screen for PD-1 ligands in the supernatants from a variety of cultured cells. I agreed to collaborate and provided not only the reagents but all our knowledge about PD-1 because this molecule was completely unknown to them. Meantime, we tried to identify the PD-1 ligand by fishing for molecules which bound to an engineered PD-1-Ig fusion protein. Although we clearly detected binding of PD-1-Ig protein to various cell types, including some tumor cells, we were unable to purify or identify the miniscule amount of binding proteins. After a year of silence, on October 5, 1999, Clive Wood, who was responsible for this project under Steve, sent word out of the blue that they might have identified the PD-1 ligand. On October 25, 1999, in Boston, Clive explained that their collaborator Gordon Freeman at Dana Farber identified several B7 family cDNA in a deposited data base and the Clive group found the protein encoded by one of them (clone 292) had bound to the PD-1 expressing cells we had sent from our laboratory. In Japan, we confirmed binding of the 292 protein to PD-1, and performed experiments showing its immune inhibitory function. Together, we published our identification of a PD-1 ligand (PD-L1) (Freeman et al., 2000). Just before the submission of our paper, I learned that Lieping Chen had published a paper describing the identical cDNA as a B7 family member with costimulatory activity, without knowing its receptor (Dong et al., 1999).
Meanwhile, we continued our studies aimed at treating cancer by PD-1 blockade. By September 2000, Iwai had found that myeloma expressing the PD-L1 grew more slowly in PD-1 deficient mice compared with wildtype mice. I was very excited and convinced that PD-1 would make an excellent target for cancer treatment. As I needed good blocking antibodies against PD-1 or PD-L1, I talked with Minato and urged him to hurry in our collaborative work towards this goal. Minato’s group had already started searching for antibodies which could effectively block either PD-1 or PD-L1. Yoshimasa Tanaka in Minato’s laboratory worked diligently on the project, and by the end of 2001 we had gathered enough data to demonstrate that PD-1 blockade by PD-L1 antibodies can prevent different types of tumor in mouse models (Iwai et al., 2002) (Fig. 5). In my own group, Iwai showed that the spread of B16 melanoma cancer from spleen to liver could also be prevented by anti-PD-1 antibody treatment (Iwai et al., 2005).
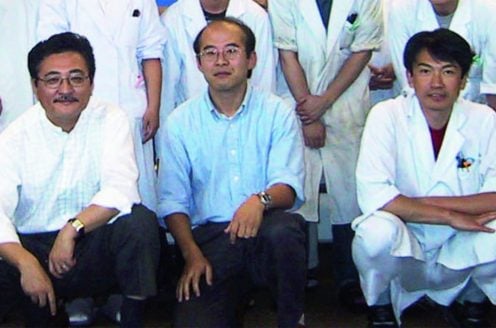
Figure 5. From left to right, Nagahiro Minato, Masakazu Hattori and Yoshimasa Tanaka in 2000.
As we prepared our publication about PD-L1 isolation, I asked Wood how we might approach patent applications, and was informed that Wood and Gordon had already applied for a patent covering PD-L1 and its usage in November 1999 without informing me. I was surprised and registered a complaint through a lawyer, but to no avail. However, Wood’s patent was declined, perhaps lacking sufficient data to support its usage. More unpleasant surprises were to come, as more than 10 years after our PD-1 blockade paper was published, Freeman and Wood sued me by claiming co-inventorship of the PD-1 cancer immunotherapy patent. The precise timelines in my story thus arise from a desire to publicly clarify how we came to discover the therapeutic value of PD-1, due to my draining and still unresolved legal battle regarding the intellectual property rights.
Clinical application
As I anticipated the clinical application of our finding on tumor treatment by PD-1 blockade, I approached Kyoto University to apply for the intellectual property right for usage of PD-1 blockade for cancer treatment. Frustratingly, they lacked the human and financial resources to support my application. At this point I turned to a small Japanese company, Ono Pharmaceutical, because we had been involved in other collaborations.
Unfortunately, Ono also had no capacity to organize clinical trials for cancer drug development and searched for a bigger partner. They spent almost a year visiting more than a dozen companies without success. It was obvious that, at this time, neither the pharmaceutical industry, clinicians, nor even many biologists believed that trying to enhance the immune system could help cure cancer. I was terribly disappointed when Ono officials told me they had decided to abandon the project. I decided to turn to my network of friends in the USA, many of whom had started their own companies.
I visited one such venture capital company in Seattle and they surprisingly agreed to invest in my proposal almost instantly, on the condition that Ono would be excluded from future developments. I waited for several months for Ono to confirm they had no intention to use our patent independently before committing to the Seattle partner. Ono then stunned me with the news that they now had the resources to invest in developing a PD-1 treatment. Later, I learned that Medarex, the biopharmaceutical company led by Nils Lonberg had found our published PCT patent application and directly approached Ono with a collaboration proposal. I was extremely lucky again. Without this chance encounter, the PD-1 cancer drug development might have been delayed by many years.
The paper summarizing the first clinical trial (Phase I) on PD-1 antibody published by Suzanne Topalian’s group in 2012, showed very promising complete or partial responses in roughly 20 to 30 percent of patients with terminal melanoma, lung, or renal cancer. In addition, patients continued in unprecedented good health for more than a year and, half after treatment. In Japan, we carried out a smaller clinical trial on drug-resistant ovarian cancer patients in collaboration with the Gynecology Department at Kyoto University. I was immensely pleased to hear about a patient whose large tumor had been completely cured by PD-1 blockade therapy, and to watch her enjoying a round of golf on a TV program about the breakthrough drug. Two patients treated for a year with PD-1 antibody are still free from tumors almost five years later. The PD-1 antibody was approved for melanoma treatment by PMDA in Japan in 2014, quickly followed by the FDA in the USA.
Science media
In 2013, Science magazine selected cancer immunotherapy as the number one scientific breakthrough of the year. The author of that article described the development of therapy targeting CTLA-4 by Jim Allison in detail. However, I was shocked as the article continued: “In the early 1990s, a biologist in Japan discovered a molecule expressed in dying T cells, which he called programmed death 1, or PD-1, and which he recognized as another brake on T cells. He wasn’t thinking of cancer, but others did.” I was speechless! Clearly, this Science editor reporting cancer immunotherapy had either not read our papers describing cancer therapy by PD-1 blockade, or had intentionally neglected them (Iwai et al., 2002, 2005).
This article was a symbol to me of deficits in our current system of science journalism. Scientists are eager to publish their papers in the so-called top journals like Science, Nature or Cell, as they are judged by their peers and funders based on such publications. When I first became a researcher, editors of these journals such as Miranda Robertson, Peter Newmark, Ben Lewin and Linda Miller, were very knowledgeable, reliable and sincerely interested in research. I was happy to send my manuscripts to their journals as I felt confident these editors had the knowledge and training to allow them to perceive the importance of our science. However, more recently, perhaps due to the enormous expansion in the number of journals, this no longer seems to be the case. When in-house editors rely on the majority vote of outside reviewers with different opinions rather than their own judgment, paradigm-shifting papers have difficulty being published, as by definition they run against the concepts held by the majority. It is notable that none of my papers cited by the Nobel Committee were published in these so-called top journals. The current peer review system tends to reject newly emerging concepts or new findings running against the established dogma in the scientific community. Since scientists feel they have at some point received unfair statements from our colleagues, I believe revealing the names of peer reviewers is one way we might mitigate some of these problems. Certainly the status quo needs to change to make our current peer review system more constructive and efficient.
Six “C”s in science
To carry out science, I always say to my students; if they do not have Curiosity, then they should not choose a career in science. There are many types of scientists. It is always important to find out what you really want to know. Imagine research as a journey deep into mountains to find the origin of the mysterious river. Sometimes on the path you may discover an interesting stone which you bring back and find it very precious upon careful examination. My style of doing science is to find something totally unknown, or to map a totally new route to an unknown cave from which water is coming out. You should keep asking what you wish to know rather than what you can do. You need to embrace Challenge and have the Courage to invest your effort and time. These three “C”s were the basis of my research career. Once you fix on a project, three more “C”s are required. Of course, you have to Continue your research with lots of Concentration. Concentration means placing research as a central activity in your life, sometimes sacrificing other important calls on your time to focus on your goal. To Continue and Concentrate, you may need Confidence that you can do it. Or it could be the other way around. Concentration and Continuation may eventually build up Confidence. The last three “C”s are as important as the first.
Dreams
Aside from scientific activities, I still have two dreams at the age of 76. As I have watched younger generations struggling for support, I have long thought Japanese universities should create their own funds to support young scientists. Compared to American universities with large endowments, Japanese universities rely more on government funds. Since the future prospects of the science budget from the Japanese government are not so promising, I have proposed that Kyoto University set up a fund using royalties from our PD-1 patent. Fortunately, this fund has already started accepting donations. I sincerely hope others will join this initiative to help instill scientific enthusiasm in the next generations of researchers. This is especially critical in Japan, where human creativity is our most important natural resource.
Another dream of mine is age-shoot. I started playing golf while living in the United States. Over there, the green fee was only five dollars, which even an impoverished post-doc could easily afford. Perhaps I love golf for its similarities to science. For every shot, the given conditions are never the same. You have to think how far you are going to hit, in which direction and whether to add spin to stop on the green and so on. Most importantly, one must avoid big mistakes. It is totally different from team sports or those where you must score points. In golf, you have to always choose from many ways to approach a shot and this decision lies with you alone. Probably, this challenging game sparked my curiosity to which I am so attached. I dream of achieving a score equal to my age before I turn 80.
Coda
The curiosity surrounding my two favorite molecules, PD-1 and AID, will continue thanks to my long-time collaborator, the Romanian scientist Sidonia Fagarasan (Fig. 3). Her work in my lab and beyond revealed that PD-1 and AID are essential molecules for the generation and selection of intestinal IgA that regulates our microbiota. Microbiota, in turn, control the immune system by promoting expression of AID, PD-1 and IgA, which are essential for maintaining immune tolerance to microbiota and self, metabolic balance and even brain function. Using AID-deficient mice, Sidonia was the first scientist to show that dysregulated gut microbiota greatly change the host immune system (Fragasan et al. 2002). How serendipitous that my two favorite molecules should collaborate in such a fascinating way to control the immune system, the microbiome and to fine-tune anti-tumor activity! It feels impossible to learn everything even just about these two molecules, as they have a far-reaching impact on other major physiological systems of the body. Indeed, despite so much progress, I believe we are just beginning our exploration of life sciences, because we understand so little about biology and physiology. After all, medicine has made great leaps in fixing acute problems, but when it comes to physiology we are humbled by the body’s complexity, as we are decoding the continuous evolutionary experiments of the past four hundred and sixty million years. Every day I come to the lab enthusiastic to learn more about immune regulation in the context of physiological systems, still dreaming that curing cancer patients everywhere on this planet by immunotherapy will become possible someday.
Acknowledgements
I am deeply grateful for the precious support for the preparation of this article by Sidonia Fagarasan, Alexis Vogelzang, Blake Thompson and Maki Kobayashi.
References
Dong, H., Zhu, G., Tamada, K., and Chen, L. (1999) B7-H1, a third member of the B7 family, co-stimulates T-cell proliferation and interleukin-10 secretion. Nature Medicine 5, 1365–1369.
Fagarasan, S., Muramatsu, M., Suzuki, K., Nagaoka, H., Hiai, H., and Honjo, T. (2002) Critical roles of activation-induced cytidine deaminase in the homeostasis of gut flora. Science 298, 1424–1427.
Freeman, G. J., Long, A. J., Iwai, Y., Bourque, K., Chernova, T., Nishimura, H., Fitz, J., Malenkovich, N., Okazaki, T., Byrne, M. C., Horton, H. F., Fouser, L., Carter, L., Ling, V., Bowman, M. R., Carreno, B. M., Collins, M., Wood, C. R., and Honjo, T. (2000) Engagement of the PD-1 immunoinhibitory receptor by a novel B7 family member leads to negative regulation of lymphocyte activation. The Journal of Experimental Medicine 192, 1027–1034.
Honjo, T., Nishizuka, Y., Hayaishi, O., and Kato, I. (1968) Diphtheria toxin-dependent adenosine diphosphate ribosylation of aminoacyl transferase II and inhibition of protein synthesis. Journal of Biological Chemistry 243, 3553–3555.
Honjo, T., and Kataoka, T. (1978) Organization of immunoglobulin heavy chain genes and allelic deletion model. Proceedings of National Academy of Sciences of the United States of America 75, 2140–2144.
Ishida, Y., Agata, Y., Shibahara, K., and Honjo, T. (1992) Induced expression of PD-1, a novel member of the immunoglobulin gene superfamily, upon programmed cell death. The EMBO Journal 11, 3887–3895.
Iwai, Y., Ishida, M., Tanaka, Y., Okazaki, T., Honjo, T., and Minato, N. (2002) Involvement of PD-L1 on tumor cells in the escape from host immune system and tumor immunotherapy by PD-L1 blockade. Proceedings of National Academy of Sciences of the United States of America 99, 12293–12297.
Iwai, Y., Terawaki, S., and Honjo, T. (2005) PD-1 blockade inhibits hematogenous spread of poorly immunogenic tumor cells by enhanced recruitment of effector T cells. International Immunology 17, 133–144.
Muramatsu, M., Kinoshita, K., Fagarasan, S., Yamada, S., Shinkai, Y., and Honjo, T. (2000) Class switch recombination and hypermutation require activation-induced cytidine deaminase (AID), a potential RNA editing enzyme. Cell 102, 553–563.
Nishimura, H., Nose, M., Hiai, H., Minato, N., and Honjo, T. (1999) Development of lupus-like autoimmune diseases by disruption of the PD-1 gene encoding an ITIM motif-carrying immunoreceptor. Immunity 11, 141–151.
This autobiography/biography was written at the time of the award and later published in the book series Les Prix Nobel/ Nobel Lectures/The Nobel Prizes. The information is sometimes updated with an addendum submitted by the Laureate.
Nobel Prizes and laureates
Six prizes were awarded for achievements that have conferred the greatest benefit to humankind. The 12 laureates' work and discoveries range from proteins' structures and machine learning to fighting for a world free of nuclear weapons.
See them all presented here.