Burton Richter
Biographical

I was born on 22 March 1931 in New York, the elder child of Abraham and Fanny Richter. In 1948 I entered the Massachusetts Institute of Technology, undecided between studies of chemistry and physics, but my first year convinced me that physics was more interesting to me. The most influential teachers in my undergraduate years were Professors Francis Friedman, who opened my eyes to the beauty of physics, and Francis Bitter, who gave me my first opportunity to do serious experimental physics.
In the summer following my junior year, I began work with Bitter in MIT’s magnet laboratory. During that summer I had my introduction to the electron-positron system, working part-time with Professor Martin Deutsch, who was conducting his classical positronium experiments using a large magnet in Bitter’s laboratory. Under Bitter’s direction, I completed my senior thesis on the quadratic Zeeman effect in hydrogen.
I entered graduate school at MIT in 1952, continuing to work with Bitter and his group. During my first year as a graduate student, we worked on a measurement of the isotope shift and hyperfine structure of mercury isotopes. My job was to make the relatively short-lived mercury-197 isotope by using the MIT cyclotron to bombard gold with a deuteron beam, a kind of reverse alchemy. By the end of the year I found myself more interested in the nuclear and particle physics problems to which I had been exposed and in the accelerator I had used, than in the main theme of the experiment. I arranged to spend six months at the Brookhaven National Laboratory’s 3-GeV proton accelerator to see if particle physics was really what I wanted to do. It was, and I returned to the MIT synchrotron laboratory. This small machine was a magnificent training ground for students, for not only did we have to design and build the apparatus required for our experiments, but we also had to help maintain and operate the accelerator. My Ph.D. thesis was completed in 1956 on the photoproduction of pi-mesons from hydrogen, under the direction of Prof. L.S. Osborne.
During my years at the synchrotron laboratory, I had become interested in the theory of quantum electrodynamics and had decided that what I would most like to do after completing my dissertation work was to probe the short-distance behavior of the electromagnetic interaction. At that time renormalization was not yet part of the theory bag of tricks, and many talked of the possibility of a high energy cutoff to the electromagnetic force. I wanted to see if the cutoff was real, and, if so, at how small a distance it came into force. So I sought a job at Stanford’s High-Energy Physics Laboratory where there was a 700-MeV electron linear accelerator. My first experiment there, the study of electron-positron pairs by gamma-rays, established that quantum electrodynamics was correct to distances as small as about 10-13 cm which was a new limit on its range of validity.
In 1957, G.K O’Neill of Princeton proposed building a colliding beam machine that would use the HEPL linac as an injector, and allow electron-electron scattering to be studied at a center-of-mass energy ten times larger (or a distance ten times smaller) than my pair experiment. I joined O’Neill, and with W.C. Barber and B. Gittelman, we began to build the first colliding-beam device. It took us about six years to make the beams behave properly. This device was the ancestor of all of the colliding-beam storage rings to follow. The technique has been so productive that all high-energy physics accelerators now being developed are colliding-beam devices.
In 1960, I married Laurose Becker. We have two children, Elizabeth, born in 1961, and Matthew, born in 1963.
In 1965, after we had finally made a very complicated accelerator work and had built the needed experimental apparatus, the experiment was carried out, with the result that the validity of quantum electrodynamics was extended down to less than 10-14 cm.
Even before the ring at HEPL was operating, I had begun to think about a high-energy electron-positron colliding-beam machine and what one could do with it. In particular, I wanted to study the structure of the strongly-interacting particles. Robert Hofstadter had studied the structure of the proton with electron-proton scattering (for which he was awarded the Nobel Prize in 1961), and, in principle, it was possible to get a related picture of the structure of unstable particles such as mesons with electron-positron colliding beams.
I had been thinking about how to do it when in 1963 Professor W.K.H. Panofsky invited me to come to the Stanford Linear Accelerator Center (SLAC). I accepted, and, with his encouragement, I set up a group to make a final design of a high-energy electron-positron machine. We completed a preliminary design in 1963 and in 1964 submitted a formal request for funds to the Atomic Energy Commission. That was the beginning of a long struggle to obtain funding for the device, during which I made some excursions into other experiments. My group designed and built part of the large magnetic spectrometer complex at SLAC and used it to do a series of pi- and K-meson photoproduction experiments. Throughout this time, however, I kept pushing for the storage ring and kept the design group alive. Finally, in 1970, we received funds to begin building the storage ring (now called SPEAR) as well as a large magnetic detector that we had designed for the first set of experiments. In 1973 the experiments finally began, and the results were all that I had hoped for. The discovery for which I was honored with the Nobel Prize and the experiments that elucidated exactly what that discovery implied are described in the accompanying lecture. Much more has been done with the SPEAR storage ring, but that is another story.
I spent the academic year, 1975-76, on sabbatical leave at CERN, Geneva. During that year I began an experiment on the ISR, the (CERN 30 by 30 GeV proton storage rings), and worked out the general energy scaling laws for high-energy electron-positron colliding-beam storage rings. My motive for this last work was two-fold: to solve the general problems and to look specifically at the parameters of a collider in the 100-200 GeV center-of-mass (c.m.) energy range that would, I thought, be required to better understand the weak interaction and its relation to the electromagnetic interaction. That study turned into the first-order design of the 27-km circumference LEP project at CERN that was so brilliantly brought into being by the CERN staff in the l980s.
An interesting sidelight to the LEP story is the attempt by Professor Guy von Dardel (then at Lund), Chairman of the European Committee for Future Accelerators, and I to turn LEP into an inter-regional project. We failed because we couldn’t interest either the American or European high-energy physics communities in collaboration even on as large a scale as LEP. The time was not right.
The general scaling laws for storage rings showed that the size and cost of such machines increased as the square of the energy. LEP, though very large, was financially feasible, but a machine of ten times the energy of LEP would not be. I began to think about alternative approaches with more favorable scaling laws and soon focused on the idea of the linear collider where electron and positron beams from separate linear accelerators were fired at each other to produce the high-energy interactions. The key to achieving sufficient reaction rate to allow interesting physics studies at high energies was to make the beam extremely small at the interaction point, many orders of magnitude less in area than the colliding beams in the storage rings.
In 1978 I met A.N. Skrinsky of Novosibirsk and Maury Tigner of Cornell at a workshop we were attending on future possibilities for high-energy machines. We discovered that we had all been thinking along the same general lines and at that workshop we derived, with the help of others present, the critical equations for the design of linear colliders. On returning from the workshop I got a group of people together at the Stanford Linear Accelerator Center and we began to investigate the possibility of turning the two-mile-long SLAC linac into a linear collider. It would be a hybrid kind of machine, with both electrons and positrons accelerated in the same linear accelerator, and with an array of magnets at the end to separate the two beams and then bring them back into head-on collisions. The beams had to have a radius of no more than two microns at the collision point to get enough events to be interesting as a physics research tool, roughly a factor of 1000 less in area than the colliding beams in a storage-ring collider like LEP.
Construction of SLAC Linear Collider began in 1983, and was finished in late 1987. The first physics experiments began in 1990 after a difficult start up. This was a new kind of accelerator complex, and, though we had anticipated some of the new problems we would face, we had not anticipated all. The staff of SLAC did overcome them all and by the time the machine was turned off to make way for the next lab facility, the machine was colliding polarized electrons with positrons at an interaction point where the beam area was a factor of 20 smaller than the original design.
While some important experiments were carried out, probably the most lasting contribution that this facility made to particle physics was the work on accelerator physics and beam dynamics that was been done with the machine and which forms the basis of a very active world wide R&D programs aimed at TeV-scale linear colliders for the future. This R&D program is being pursued in the U.S., Europe, and Japan. This will be the inter-regional machine that von Dardel and I tried to make of LEP in the later 1970s.
Along the way I succumbed to temptation and became a scientific administrator, first as Technical Director of the Stanford Linear Accelerator Center from 1982 to 1984, and then Director from 1984 to 1999. The job of a laboratory director is much different from the job of a physicist, particularly in a time of tight budgets. It is much easier to do physics when someone else gets the funds than it is to get the funds for others to do the research.
While a lab Director can get done the things that he regards as important, he has the more important job of bringing out the best ideas of the broader scientific community. I learned this early in my career while I was leading the construction of the SPEAR facility. During the construction process I was approached by two Stanford faculty, Sebastian Doniac and William Spicer, who wanted to use the intense x-rays produced in the colliding beam facility to do work in condensed matter physics. Their point was that it was possible to make x-ray beams a million times more intense than could be achieved with x-ray tubes, and with such intensity it would be possible to do revolutionary experiments. They were convincing and, since the world was simpler then than now, I just did it, spending the extra funds required to make a port in the machine to let the beams out. This was the first of the modern synchrotron radiation facilities and it did what they promised. It is still in operation with many other such facilities for which it served as the prototype.
Modern science is fast-moving and no laboratory can exist for long with a program based on old facilities. Innovation and renewal are required to keep a laboratory on the frontiers of science and only if it remains on the frontiers will it have a long-term future. Developing the idea of the community to the point where the best of them are ready for implementation requires significant resources. It is important that these resources be made available even in times of tight budgets. Starving the future to feed the present is a mistake – it leads to obsolescence and stagnation. Sometimes it is hard to make this understood.
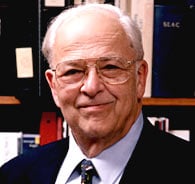
During my time as Director the synchrotron radiation program was integrated into the laboratory and the development of an x-ray free-electron laser, based on the linear-collider technology, was begun. It is today (2005) under construction.
SLAC also began to move into astro-particle physics with the international collaboration know as GLAST (gamma-ray, large area, space telescope). The astro-particle program has continued to grow under the new Director.
I also began an interregional collaboration with my counterparts in Europe (Bjorn Wiik at the DESY lab in Germany) and Asia (Hirotaka Sugawara at the KEK laboratory in Japan) aimed at bringing into existence a high-energy linear collider as a world collaboration. There is now a consensus that this is the right thing to do and a global organization is being created to bring it to reality. Perhaps von Dardel and my dream will now become real.
Since stepping down as laboratory director in 1999 I have devoted an increasing fraction of my time to international issues. I am involved with energy, environment, and sustainability issues, particularly as they involve new energy sources free of greenhouse gases. Getting enough energy to satisfy the needs of the developing world without bringing on an eco-disaster is not going to be easy. It will require a marriage of science and technology with good international policy, something that is always hard to bring off. We need to get it right this time.
* This autobiography was provided by the Laureate in May 2005.
Burton Richter died on 18 July 2018.
Nobel Prizes and laureates
Six prizes were awarded for achievements that have conferred the greatest benefit to humankind. The 12 laureates' work and discoveries range from proteins' structures and machine learning to fighting for a world free of nuclear weapons.
See them all presented here.