Saul Perlmutter
Biographical
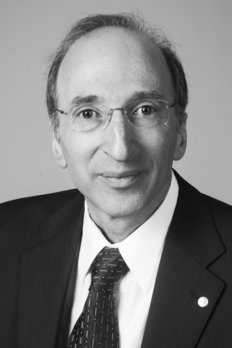
My four grandparents all immigrated as young adults to the United States from Eastern European Jewish towns and villages early in the twentieth century. This was a generation of poor but optimistic intellectuals, who expected that the newly rationalist world would use education and creativity to leave behind boundaries, borders, and religion to build a better world. (My mother’s father, for one, although he earned a living welding in the shipyards during the Second World War and later ran a sandwich stand, was by avocation a self-educated scholar and teacher of Yiddish literature and history.) It is perhaps not surprising then that their only children, my parents, both became professors, my mother, Felice Davidson Perlmutter, in Social Work and Social Administration, and my father, Daniel D. Perlmutter, in Chemical Engineering.
My mother’s work life was full of research collaborations that she organized and enjoyed professionally and personally. The social work field appeared full of warm, friendly people who took great pleasure in working with each other. My father’s work involved careful, accurate calculations with slide rule and graphs (both attractive to me as a child), and experiments with lab equipment – and graduate students whom he taught patiently and mentored. On weekends our home was full of my parents’ friends, discussing politics and movies, books and arts late into the night. Concerns about social and political conditions of the world were a constant theme.
In this atmosphere, I grew up wanting to know all the “languages” – music, literature, math, science, symbols, architecture, psychology – particularly those that seemed most universal. I thought everybody needed to know what was in the missing “Owner’s Manual” of the Universe, since we lived here and “used” it. The Quaker schools my two sisters, Shira and Tova, and I attended encouraged creativity, critical thought, and social concerns; there were excellent teachers in the basic math and science courses but (at the time) not much beyond the fundamentals to encourage further creativity in these areas. Instead, I was excited to learn to write clearly and expressively, and to become familiar with the non-science approaches to the world.
As with many scientists, music was an important part of my life. I don’t know if there is a documented relationship between musical and scientific thinking, but there certainly seem to be shared elements, perhaps starting off with the delayed gratification inherent in practicing an instrument. In my case, my violin teacher Frances Duthie (the only teacher who was a constant throughout my school years) certainly taught a strong ethic of perfectionism, but in the service of a warm, humanistic tradition of interpersonal communication, and the chamber music ideal of collaborative, shared listening and contributing. The pleasure of group music-making continued though string-quartet playing and group singing throughout my life – and my favorite science group experiences share some of the same feeling. (Many years later, as a professor at Berkeley, I was glad to have the opportunity to design a “Physics & Music” course for undergraduates.)
By the time I reached college, I thought I would follow my fascination with the big Philosophical Questions. As I saw it, the two big mysteries are: How does the world work? and How does the mind work? (The latter was also relevant to the former, since we only perceive the world through our minds.) So starting Harvard, in 1977, I considered double majoring in Philosophy and Physics – until I realized that if I did this there would be no time for courses in Humanities and Social Sciences. I decided to major in Physics first, since it seemed more likely that I would later come back to Philosophy than the other way around. (We’ll see.)
College then became a chance to take courses in many fields. I discovered a strong interest in the then relatively-new area of Cognitive Sciences. I found out that Biology was not the boring field full of memorization that I had taken it to be, but in fact new exciting concepts were being discovered every day. I learned that Physics is a very social activity, with groups of friends and roommates meeting every week to solve the math and physics problems sets. I also found that there is barely any time to digest the implications of the physics that you learn as an undergraduate – and when I graduated in 1981 I was curious to go on to graduate school and have a chance finally to focus on the physics.
I chose to go to the University of California, Berkeley, for graduate school because I wanted a wide variety of strong experimental research groups to choose from, and this was something that Berkeley appeared to offer well beyond the other top physics departments. When I started grad school, my goal was to find a research project with real data (not just theory) that would address a deep philosophical question. The most likely route for this was a particle physics accelerator experiment, and I expected to end up doing this; but first I thought I should look to see if I could learn on a more small-group project. In my second year was lucky enough to find an unusual, dynamic, eclectic research group led by Professor Richard Muller.
Rich followed – perhaps outdid – the tradition of his mentor Luis Alvarez to pursue interesting research topics wherever they arose, with a can-do experimentalist’s attitude – and rigorous skepticism of all claims. When I joined the group, the group members were reporting at the weekly meeting on their work: (1) an idea for a new fundamental physics experiment, measuring the gravitational deflection of starlight by Jupiter; (2) a robotic-telescope supernova search to measure the Hubble constant; (3) a Raman scattering measurement of atmospheric carbon to study the carbon cycle; (4) a table-top cyclotron for radioisotope dating; (5) cosmic microwave background measurements searching for anisotropy; and (6) sundry topics, including implications of the impact theory that Luis had recently developed with his son to explain the extinction of the dinosaurs. This was the tradition of physicists at their best: playing with ideas, building toys. (Caution: physicists at play!)
I soon focused on the robotic-telescope supernova project, since it seemed to offer the possibility of a fundamental measurement, the Hubble constant. I ended up developing the software (and a little hardware) that made it possible to automatically identify the supernovae in the images, and rule out sources of confusion such as asteroids and cosmic rays. I had always enjoyed playing with computers, and the latest computers were just getting fast enough and with enough memory to make possible the near-real-time image analysis that triggered the follow-up of the supernovae. By the time I graduated with my PhD in 1986 the automated supernova search was successfully up and running, and I was asked to stay on as a postdoc to get the results from this project. (Along the way, I had of course been drawn into the myriad topics of Rich’s group, and my thesis actually used the same robotic telescope and image analysis techniques to search for a possible companion-star of our Sun, which had been proposed to explain periodic mass extinctions on the earth every 26 million years – the extinction of the dinosaurs was just one of these.)
In 1980 when Rich had begun the Hubble constant project, it looked likely that the so-called Type II supernovae would be used as a distance indicator for the measurement, with a calibration based on the Baade-Wesselink expanding photosphere method (later studied by my co-Laureate Brian Schmidt). But by the time the project was up and running in 1986, there was evidence (presented particularly strongly by Gustav Tammann and his student Bruno Leibundgut) that the new sub-classification of Type “Ia” supernovae could be used as an alternative (perhaps better) distance indicator. This news prompted Dr. Carl Pennypacker (a more senior researcher in Rich’s group) and me to think about possible implications for new projects. The Type Ia supernovae were significantly brighter than the average Type II, so they could in principle be studied at much further distances. Ever since the first supernovae were studied in the 1930’s there had been the hope that they could someday be used to measure the deceleration of the universe’s expansion – now the uniformity of the new Type Ia subclassification opened up the possibility that this idea could be revisited. And now we also had several new tools at our disposal to exploit the Type Ia supernovae: for the robotic supernova search we had worked with the very first generation of “charge-couple-device” (CCD) detectors to be used for ultra-sensitive astronomical imaging, and we had developed the image analysis tools that could sift through the large amounts of digital data that the CCD’s produced.
Late in 1987 Carl and I thus proposed a new project: we would design and build a new wide-field camera, the widest ever with a CCD on a 4-meter class telescope, and develop the software to search through tens of thousands of galaxies in one night. Unlike our previous robotic nearby supernova search, which studied one galaxy in each image, this approach would allow us to look at thousands of much more distant galaxies at a time. We estimated that in several years we could in this way discover sufficient numbers of supernovae at redshifts as high as ~0.3 that we could make an excellent measurement of the deceleration parameter. The project got off the ground in 1988 with the support of our skeptical mentor, Rich, and was one of the founding projects of Berkeley’s new Center for Particle Astrophysics.
The project began slowly with only two-and-a-half nights of good weather out of more than a dozen nights scheduled over almost two years at the Anglo-Australian 4-m Telescope in Siding Springs, Australia. Still, by 1992, when I was asked to take over from Rich as leader of the supernova research group, we had found a Type Ia supernova at z = 0.45 – doubling the world’s high-redshift sample. This was at the time the highest redshift supernova known. (The other high-redshift type Ia SNe was the one found in a several year search by a Danish team led by Norgaard-Nielson.) In the next two years, we parlayed this success into access to other large telescopes (with somewhat more reliable weather).
Two key problems stood in our way: relating brightnesses of high- and low-redshift supernovae (measured in different filters); and guaranteeing distant supernova discoveries in advance – and in time to measure their peak brightness. Without such a guarantee, one could not obtain time on the large telescopes needed to study them. By 1994, we had solved these problems and we were able to guarantee entire “batches” of multiple high-redshift supernovae, all still brightening, and all found on a pre-selected date, perfect for scheduling the measurements of brightness and spectrum. Such “guarantees” led us to propose a novel use of the Hubble Space Telescope: precision measurements of distant supernovae, particularly important for the ultra-far z~1 supernovae that collaborator Ariel Goobar (then a postdoc in Berkeley, now a Professor at Stockholm University) and I had shown could be used to distinguish among cosmological theories.
Meanwhile, between 1990 and 1993 several approaches had been developed to further calibrate the Type Ia standard candle: David Branch showed that selecting by color could give a standardized set, while Mark Phillips showed that a relationship could be established between the peak luminosity and the timescale of the brightening and fading lightcurve. A beautiful dataset of low-redshift supernovae had been found in the Calan/Tololo supernova search (led by Mario Hamuy, Jose Maza, Mark Phillips, and Nick Suntzeff) that allowed these improved calibrations to be made. So, by late 1994, after establishing that we had an effective approach with our batch discovery and multi-band follow-up of high-redshift supernovae, our now-international team of scientists was working together round-the-clock, collecting new batches of high-redshift supernova data using the best telescopes in the world for the purpose. And so was a new, competing team of experienced supernova researchers, organized by co-Laureate Brian Schmidt.
Finally, in 1997, we were analyzing our haul of 42 Type Ia supernovae at redshifts about z~0.5 and finding an odd result: the universe’s expansion was apparently dominated by a cosmological constant, or more generically a “dark energy” pervading all space, so it was actually speeding up – this didn’t fit with known models of physics! We announced this startling evidence for a cosmological constant at the American Astronomical Society January 1998 meeting. Because both our team and Brian’s team -including co-Laureate Adam Riess – independently announced matching results at conferences in the beginning of the year, by the end of the year most of the scientific community had accepted the extraordinary findings.
When we started the project we thought that whatever answer we found would be exciting: if the universe were decelerating enough we would know that the universe is finite and is coming to an end in a Big Crunch; if not then we could establish that the universe is likely infinite in space and time, and the inflation theory would have a successful prediction. We could not have hoped for the actual outcome, a surprise that presents a new puzzle to fundamental physics. This is the sort of conclusion to a project that in turn initiates many new projects. We now have the fun of trying to figure out what it is that causes the universe to accelerate. Since 1999 I have been working with colleagues on such new projects, including the development of a new space telescope that can make a much more precise measurement of the expansion history of the universe.
Perhaps when my wife, Laura Nelson, and I send our now eight-year-old daughter, Noa, to college, science will have the next installment of answers – or, better yet, new surprising questions about our world.
© The Nobel Foundation 2019
This biography was submitted in June 2019.
Nobel Prizes and laureates
See them all presented here.