Barry C. Barish
Biographical
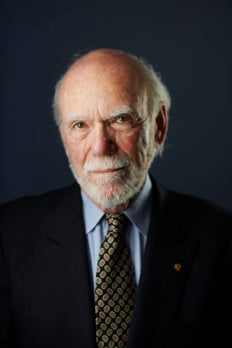
R
eflecting on my background, it is not at all apparent why my life did not go in other directions, rather than my becoming a physicist, and one dedicated to an academic career and pursuing research on some of the most fundamental problems in nature. My families’ backgrounds are not very well documented, but I do know that both my parents’ families immigrated to the U.S. from small shtetls in Eastern Europe, in the general area of Ukraine and Belarus. I know little about my ancestors there. They apparently were not very distinguished, and I do not know what they did or under what circumstances they immigrated to the U.S. at about the turn of the 20th century.
My mother’s family (maiden name Shames) settled in St Joseph, Missouri, where she was born. They moved while she was a child to Omaha, Nebraska, where her father, Max, opened a body and fender shop, where they fixed cars. My mother had one sister and they lived a middle-class life with their social life centered around the small, but tight knit, Jewish community in Omaha. My grandmother died at a young age and my grandfather, Max, remarried. My mother graduated from high school and had aspirations to go to college at the University of Nebraska, even earned a scholarship, but her father would not let her go. This was a disappointment she expressed her whole life.
My father’s father, two brothers and grandfather immigrated to the U.S. in 1901, and became homesteaders in North Dakota, under a program where the U.S. government gave away land to be farmed and developed. They did this for 18 months, sold the land for a good profit and moved to Sioux City, Iowa, where they eventually owned and ran a Ford agency until they had a falling-out with Henry Ford over his anti-Semitic attitudes. In 1929, just before the U.S. depression, the Barish family moved to Omaha, Nebraska.
My grandfather had died in Sioux City when my father was only 10 years old. Although his mother remarried, my father had to work from a young age to help support the family. As a result, my father also never went to college.
I was born in Omaha in 1936 and we spent the first 10 years of my life there. I had a brother, born in 1940. I was a quiet child, but a very good student from a young age. My father worked for his father-in-law in his automobile repair business. During World War II, my father worked at a factory near Omaha that built military bombers for the war. He was in charge of an assembly line. As a result of his war work, he did not serve in the military during the war.

Figure 1. Photo as a young boy.
After the war, my father did not want to work any longer for his father-in-law and, instead, decided to move the family to California, where he would work for his uncle. His uncle had moved his car business to Los Angeles. We moved to the Los Feliz area, a quiet neighborhood adjacent to Hollywood. I went to public schools through high school. As a young child, I loved to read and after consuming many mystery and science fiction books and I began reading good literature, including the classics. In my young teenage years, in addition to liking story-telling, I enjoyed writing. When was asked what I wanted to do when I grew up, it was to become a novelist. At this age, I did not have any special interest in science or mathematics but was always very good in math and won some contests testing skills.
I actually spent most of my non-school hours playing sports − football, basketball and tennis. Most of my friends were made through sports. By the time I entered high school, my sports interests focused on tennis and I played on my high school tennis team for the next three years, during which time, I won some honors.
Academically, I was always at or near the top of my class, but I had no real direction. Neither of my parents had gone to college, but they were convinced of the importance of education and were committed to my brother and me getting a college education. However, they were not able to give us much guidance in terms of what we might study. I also never happened to have a good mentor in high school and, as a result, I had little idea what to study. There was a general expectation in my family that my brother and I would become either doctors or lawyers. I was not very interested, but my brother did become a lawyer!
Lacking much good guidance, I decided I would study engineering, based on the fact that I had good math skills and liked what I knew about engineering. I entered the University of California, Berkeley, where as a Freshman I took two Engineering courses, Inorganic Chemistry, Physics, Mathematics and a French Literature course. This was my first physics course, because we did not have one in my high school, and I began my love affair with physics, immediately. At the same time, my engineering courses, drafting and surveying were too tedious for me. So, I switched to becoming a physics major, not really understanding what it meant to be a physicist.
I loved Berkeley from the beginning. I thrived in the academic part, made many new friends, including having my first serious girlfriend. I emerged from being a terribly shy young boy to being a socially (if not outgoing) active college student. My only disappointment was that I dropped tennis. I had aspirations to play tennis at Berkeley, but I was only marginally good enough to be on the tennis team, and the large demands for practice time interfered with my many labs in physics, chemistry and engineering.
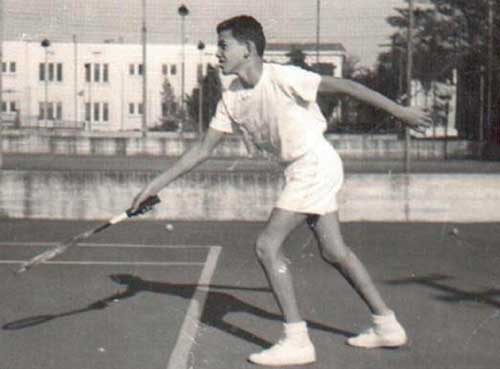
Figure 2. My days as a tennis star.
In undergraduate school, I became interested in particle physics, stimulated by all the new elementary particles being discovered on the particle accelerators at the “Rad Lab” (now Lawrence Berkeley National Laboratory, LBNL) above the campus. I did some research there as an undergrad and spent time at the 184-inch cyclotron. As I approached getting my BA, I was very attracted to doing my graduate work in Berkeley on the large accelerators. The physics department discouraged their own undergrads from going to graduate school in Berkeley, but in the end they accepted me to their graduate school.
In graduate school, after my course work and candidacy exams, I went into particle physics and did my thesis on the 184-in cyclotron studying single pion production in pion-proton collisions tracing the production of the Δ33 resonance in the final state. My thesis advisor was A. Carl Helmholz, who was chairman of the physics department. During this period I learned not just about particle physics detectors, but also the accelerators in Berkeley, the 184-in Cyclotron and the Bevatron. In fact, when I received my PhD, my wife had a job she liked and we decided to stay for an extra year, me on a postdoctoral appointment working at the Bevatron.
My years in Berkeley were transformative. I entered as a shy young boy, good academically, but otherwise pretty lost. I found and developed the professional love of my life, physics, as an undergraduate in Berkeley. I did well enough in graduate school to land a really good postdoctoral job at California Institute of Technology (Caltech), which has been my professional home ever since. I also met the other love of my life, my wife Samoan, in Berkeley and we have made our lives together ever since, she as a psychoanalyst, me as a scientist, and with a daughter and son, and three grandchildren. I left Berkeley with happiness and confidence to take on the world!
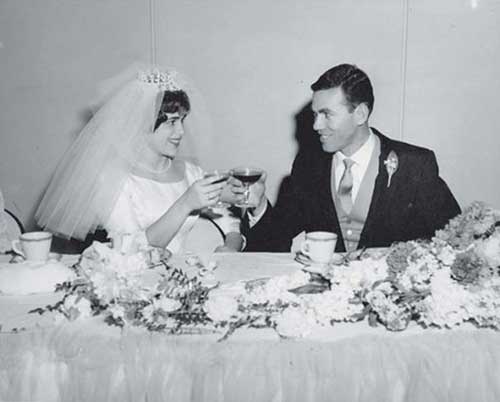
Figure 3. Marriage to Samoan in 1960.
I was hired at Caltech in the fall of 1963 as a postdoc for Alvin Tollestrup to work on a new experiment at the Brookhaven Alternating Gradient Synchrotron to study the annihilations of antiproton-proton → electron-positron pairs. This reaction probed the time-like form factors and we were searching for heavy resonances in that system, but found none. For the experiment, I designed and built an intense separated anti-proton beam as my primary contribution to the experiment. The experiment worked well and we made important time-like measurements of the proton structure for the first time. For me, it provided for the first-time, my identification with one of the major experiments in particle physics.
Then, in about 1965, I went to work on the new two-mile long linear electron accelerator at the Stanford Linear Accelerator Center (SLAC), working with Henry Kendall, Jerry Friedman of Massachusetts Institute of Technology (MIT) and Richard Taylor of SLAC. This long-term project involved designing and building a large 6-GeV spectrometer and preparing a three part program: 1) to measure the proton form factors at high momentum transfer; 2) to make a comparison of electron and positron scattering to probe two-photon exchange effects; 3) and finally, to measure electron proton inelastic scattering. These were the greatly anticipated initial experiments to be performed at the new SLAC accelerator. I participated in both the electron elastic scattering and electron-positron comparisons − highly anticipated and important studies of the proton form-factors to large momentum transfer. We found no big surprises, and I made a fateful decision not to participate in the inelastic scattering experiment, expecting that this long-term program would again provide nice measurements, but no big surprises. How wrong I was! The experiment surprisingly (at least to me) found very large scattering cross sections, which became pivotal evidence for the quark structure of the proton. My colleagues, Kendall, Friedman and Taylor, won the Nobel Prize in 1990 for this important discovery.

Figure 4. Working with Marty Breidenbach at SLAC.
I left the SLAC experiment to return to the Brookhaven AGS for a follow-up experiment from our earlier anti-proton proton annihilation experiment this time to measure final pion production states, which had been an important background in the original experiment. I organized a small group from Caltech and collaborated with Adrian Melissinos and his group from Rochester University. It was the first experiment where I was the leader (or spokesperson). The experiment was successful in that we made a series of nice measurements of high energy antiproton-proton annihilation cross sections.
On the personal side, during this period my wife and I had two children and our lives became complicated by our trying to balance my demanding professional life that required lots of travel and a home life that involved raising small children and my wife, Samoan, pursuing her own professional life as a mental health professional. I also was promoted from post-doc to Assistant Professor at Caltech.
This was a very exciting time in high energy physics. The new high energy accelerator at the National Accelerator Laboratory (now called Fermilab) was nearing completion and proposals were being made for the initial scientific program. I teamed with my Assistant Professor colleague at Caltech, Frank Sciulli, to develop a proposal to make a narrow band neutrino beam and a detector to exploit it. The high energy protons at Fermilab provided the opportunity to produce intense high energy neutrino beams. Our experiment was approved, along with a wide-band neutrino beam proposal by David Cline, Al Mann and Carlo Rubbia.
We succeeded in measuring the first high energy scattering distributions and cross sections for both neutrinos and antineutrinos. These results were complementary to the SLAC electron deep inelastic scattering experiments in revealing the quark structure of the proton. But, even more importantly, Electroweak Symmetry Breaking or the, so-called, Standard Model for particle physics had been proposed by Sheldon Glashow, Stephen Weinberg and Abdus Salam. A unique prediction of that model was that it predicted the existence of weak neutral currents, or in other words, neutrino scattering without charge exchange (v + N → v + X). An event had been observed in the Gargamelle heavy liquid Bubble Chamber at the European Organization for Nuclear Research (CERN) that provided initial evidence for neutral currents, and our experiment at Fermilab provided the definitive proof. This was the lynchpin that established the Standard Model of particle physics, still the best description of high energy physics that we have today.
In the subsequent years, I was promoted and settled into having my career based at Caltech. Interestingly, I had considered going to Caltech as an undergraduate, also as a graduate student, then I finally went as a postdoc, and have made my entire career since then at Caltech. It has been a great place to me and for me! The smallness and dedication to being a place where great science is done has provided the encouragement and support for me to address the most fundamental problems experimentally possible at the different stages of my career. I have led a series of forefront particle physics experiments, including: the Neutrino Experiment at Fermilab (with Frank Sciulli), where we established the weak neutral current; definitive evidence for the Z0, the carrier of the weak neutral current at the SLAC Linear Collider; measurements of the fundamental parameters of the t-lepton (our heaviest lepton) at the Cornell Electronic Storage Ring (CESR) electron accelerator; the most sensitive search for Grand Unified magnetic monopoles at the Gran Sasso Laboratory in Italy in the MACRO experiment, as well as experimental confirmatory evidence for the existence of atmospheric neutrino oscillations discovered by Takaaki Kajita and collaborators in the Super-Kamiokande experiment in Japan.
In 1990, I joined with Bill Willis from Columbia to co-lead the design of one of the two large efforts (Gammas, Electrons, Muons = GEM) for the Super Collider in Texas. We did detector development R&D and simulation work to optimize a detector for the main particle physics goals in the multi-TeV range. The GEM collaboration was highly international and we developed a detector design that was very well reviewed shortly before the Superconducting Super Collider (SSC) was cancelled by Congress in the fall of 1993. This was a huge disappointment for those of us in particle physics in the U.S. A large contingent of my collaborators in GEM joined the CERN Large Hadron Collider (LHC) experiments, and both the U.S. Department of Energy (DoE) and National Science Foundation (NSF) approved funding for U.S. participation at CERN. In fact, some central design features and technologies from GEM have been implemented in the LHC detectors.
Following the cancellation of the SSC, I made a personal decision not to join the CERN experiments, but was not in a rush to decide what I wanted to do next. Being a professor at Caltech, I had no job issue and my main preoccupation in the months immediately following the SSC cancellation was finding jobs for some of the very talented scientists I had hired at the SSC and who now had no job. Some of them were absorbed in the broader U.S. high energy physics program, some left high energy physics for technical jobs or Wall Street or elsewhere, and some were unfortunately lost to science.
About two months after the SSC cancellation, I received a phone call from Charles Peck, the chair of Physics, Math and Astronomy at Caltech. Charlie was a long-time colleague and friend, who I had worked with on and off through the years. He asked if he could come out and visit me in Santa Monica, where we live (about an hour drive from Caltech) to take a walk with me on the beach. This was completely out of character for Charlie, and I had no idea what he wanted to talk about (maybe to commiserate over the demise of the SSC?), but I didn’t ask, just set a time for him to come. Charlie came and we took a walk during which he asked me if I would be willing to take over the leadership of the Laser Interferometer Gravitational-Wave Observatory (LIGO). This was completely out of the blue and a surprise to me. Charlie explained the problems that existed in LIGO and that NSF wanted a change of leadership, before they would consider funding the project. He told me that the NSF Director, the MIT and Caltech Presidents, etc. had conferred and they wanted him to ask me to take the project over. I told Charlie that I was flattered, supported Caltech’s and MIT’s initiative toward gravitational wave detection, but that I hadn’t yet considered what I wanted to do next. I said that to decide on LIGO, I would need a month to do my homework to determine whether I thought I could make LIGO a success. After a month, I was not able to convince myself that I could succeed, but on the other hand, I couldn’t convince myself I couldn’t. As a consequence, I accepted and became the LIGO Principal Investigator, and I plunged into this great challenge.
In the spring of 1994, we revised the LIGO proposal in ways that raised the costs substantially. The reasons were to strengthen the technical team, have larger staffs at the sites to operate LIGO, and to invest more in making the technical infrastructure as robust, flexible and forward-looking as was practical. We made some significant design changes, including investing in some modern developing technologies like solid state lasers, digital controls, computer interferometer simulations, etc. The revised proposal was submitted to the NSF and was reviewed by a distinguished panel by summer of 1994. The new proposal was strongly endorsed by the review committee.
The next step was for NSF to make a decision whether to fund our proposal. We had strong support in Physics from Rich Isaacson and Dave Berley, who were responsible for the NSF gravity program and for LIGO and from the physics leadership, Marcel Bardon and Bob Eisenstein. The Math and Physical Sciences Assistant Director at that time was Bill Harris. I met with this group several times over this transition period. Bill became convinced that LIGO was potentially a transformative project and that we were on a path to succeed.
Bill Harris convinced Neal Lane, Director of the NSF at that time and the National Science Board (NSB) chairperson that Kip Thorne and I should be invited to make a presentation directly to the NSB, to help them be able to make an informed decision whether to fund and proceed with LIGO. Kip Thorne and I were invited to a meeting of the NSB during the summer of 1994. I note that it was unprecedented for scientific proposals to be presented to the NSB by the proposers. In any case, the NSB agreed and Kip made an inspired presentation on the science potential of LIGO. In my opinion, this made funding LIGO irresistible, if the board could only be convinced the investment would be used in a way such that the project could succeed. I followed Kip’s talk by laying out our plan for how we would build and evolve LIGO over the coming decade, as well as how to have a clear path in the longer term to Advanced LIGO, a yet more sensitive detector. The NSB accepted our arguments and approved funding to construct LIGO.
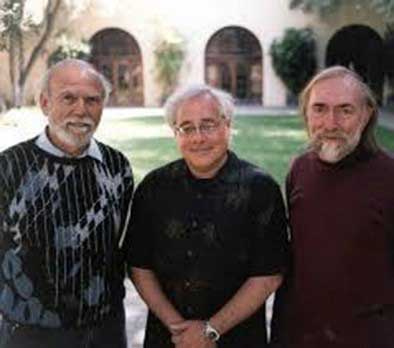
Figure 5. Gary Sanders (center), Kip Thorne and myself (left) in 2000.
We then organized the LIGO project and began to move toward construction. The project was (and is today) a Caltech-MIT collaboration, funded through a “Cooperative Agreement” between NSF and Caltech, and with MIT as a subcontractor. Although administratively MIT is a subcontractor, in fact operationally LIGO and Advanced LIGO were built and are operated by the LIGO Laboratory, consisting of staffs and Caltech, MIT, Hanford Washington and Livingston Louisiana. Some other university groups participate in particular technical areas under subcontracts with the LIGO Laboratory and non-U.S. collaborators thorough memorandums of understanding (MOUs).
The construction of Initial LIGO took place between 1994 and 1999. Some key members of LIGO who joined the project early during the construction period included: Gary Sanders, whom I had worked with at the SSC and who had experience with large projects, became our project manager; I recruited Albert Lazzarini from industry, and he took on the responsibility of being our systems engineer and integration manager; Dennis Coyne, who became our chief engineer, and Mark Coles, who became head of the LIGO Livingston Observatory (LLO). They joined the very strong team who were working on LIGO before I took it over, including Stan Whitcomb, who has been our chief scientist; Fred Raab, who became the head of the LIGO Hanford Observatory (LHO), Mike Zucker who led LLO for a few years, David Shoemaker and Peter Fritschel, who worked with Rai Weiss on the many responsibilities assumed by the MIT group.
Two important events occurred in 1997, while LIGO was in the midst of construction.
First, I invited Benoit Mours from Laboratoire d’Annecy and a senior member of Virgo to visit Caltech on sabbatical, and during that visit he worked with LIGO scientists to agree on a data format structure that would be used by both LIGO and Virgo. This was the earliest step toward these two collaborations working together to analyze data. The MOU to collaborate between LIGO and Virgo that has led to joint authorship of our discovery paper was formalized ten years later.
The second important event that took place in 1997 was the creation of the LIGO Scientific Collaboration (LSC), which brought in a broader group than Caltech and MIT to carry out the science from LIGO. The LSC was made by design to be a separate organization from the LIGO Laboratory, an organization where LIGO Laboratory scientists and scientists from other U.S. or non-U.S. universities and laboratories would work on equal footing with other individuals and groups that joined to do LIGO science. This has evolved over 20 years into the present LSC of over 1,200 members from 18 countries. The data pipelines for the various searches, calibration of data, data quality studies, scientific computing and the responsibility for scientific papers, talks etc. are carried out. The LSC has been a great success in enabling scientists from around the world to join together to carry out the science of LIGO.
In about 2000, we began commissioning LIGO and it became more sensitive than any previous instrument within about one year. Over the next decade we went through six cycles of ‘observing,’ which we called runs S1 through S6, each taking months of data, and each searching for gravitational waves and not finding any. Each of these observational runs was at significantly better sensitivity than the previous, enabling us to set better and better limits on gravitational waves. Finally, the last data runs reached our design sensitivities. One very important feature of this period is that there we had found no serious backgrounds or rejected possible candidates for gravitational waves. This meant that we were limited by our instrument sensitivity, not backgrounds that had features that could mimic gravitational waves.
At the time I proposed our plan for LIGO to the National Science Board in 1994, I asked for support to keep the key technical experts who developed Initial LIGO to do the R&D to develop the technologies for Advanced LIGO. By 2003, we had developed the basic technological concepts for Advanced LIGO and that conceptual design was reviewed and approved by the National Science Foundation. We developed an engineering design and developed the project plan and responsibilities over the next few years. Advanced LIGO was funded and we began construction soon after completion of our S5 data runs, when we had pretty much achieved the best sensitivity possible before a major upgrade to Advanced LIGO. A few changes for Advanced LIGO were implemented immediately and a final data run we called Enhanced LIGO, S6, was taken before the major construction was undertaken for Advanced LIGO.
During much of the period of Advanced LIGO construction, I was involved part-time on LIGO. I had been recruited to lead the design of the International Linear Collider, the leading concept for the next generation particle physics accelerator. For three generations, particle physics had advanced to new higher energy regimes through the complementarity of two accelerators, a proton-proton accelerator with good rates and ability to survey, and an electron-positron accelerator with capability of doing more precise measurements. Vigorous R&D programs were carried out at the Japanese High-Energy Accelerator Research Organization Laboratory (KEK), SLAC in the U.S. and Deutsches lektronen-Synchroton (DESY) in Germany to develop a design for an electron-positron machine to complement the Large Hadron Collider (LHC) at CERN. The fundamental problem is that traditional circular machines at such high energies for electrons and positrons radiate away too much energy for a practical size machine. This motivated the development of a new type of accelerator, a linear collider.
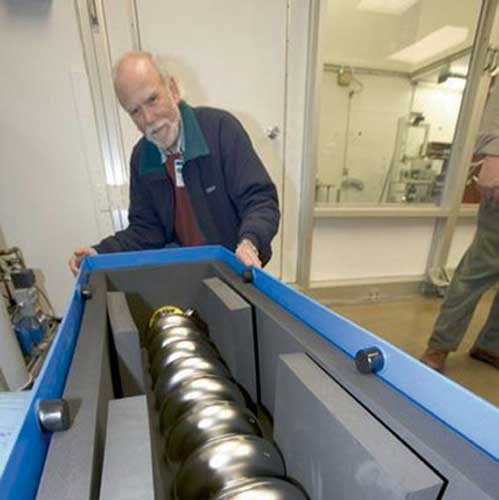
Figure 6. Inspecting superconducting cavities for the International Linear Collider.
A linear collider is a very challenging concept, because it is a single pass machine, while a conventional circular collider has many cycles for the beam particles to collide. Therefore, to achieve comparable event rate (luminosity) the beams have to be focused into very small spots. Two possible solutions were pursued in major R&D programs in the 1990s, one using conventional room temperature cavities at SLAC and KEK, and one using superconducting cavities at DESY. I chaired a committee in 2004 that chose the superconducting radiofrequency (RF) cavity concept, based to a large extent on the promise of a developing technology, other applications, and some technical advantages. I was then asked by the International Committee on Future Accelerators (ICFA) to lead the design effort for the International Linear Collider. I accepted and led an international R&D and design effort resulting in a detailed technical design that was completed in 2012 and is under consideration by the Japanese government with a decision expected during 2018.
In 2002, I was appointed by President Bush to the National Science Board (NSB). The NSB has 24 members, 8 appointed by the sitting President every two years. I served from 2002 to 2008, then as a consultant for two more years. The NSB advises both Congress and the President on science issues and serves as the oversight board for the National Science Foundation. Just as examples, during my tenure we did a study and advised Congress on the issue of ‘rare materials,’ many of which have become essential for high technology electronics, etc., yet, are not being mined in the U.S. Other issues that we grappled with continuously included diversity issues in science education and science professions. As the oversight board for the National Science Foundation we advised on how to do the best science within a system that has become more and more accountable to the government and has a smaller fraction of successful grant applications. Both trends lead to more conservatism and lack of risk taking in the NSF grant program, while doing the best science inherently involves risk taking and willingness to fail or find nothing that is interesting. My tenure on the NSB was very broadening for me, working closely with very talented and knowledgeable colleagues with very different backgrounds and skills on interesting and important issues.
I returned full-time to LIGO as the construction was complete on Advanced LIGO and commissioning was underway. The commissioning went well and when an improvement of a factor of three or more over the best achieved sensitivity for Initial LIGO was achieved, we were ready to perform the first Advanced LIGO data run. It began in September of 2015 and we observed the merger of two Black Holes on September 14, 2015. This discovery was a direct result of the new technology we had developed to improve our isolation from the ground motion by adding seismic isolation to the passive isolation in LIGO. This technology was developed in the Advanced LIGO R&D program that immediately followed the construction of Initial LIGO.
My first reaction to the dramatic event of Sept 14, 2015 was concern that we might be either fooling ourselves or were being fooled. The Advanced LIGO interferometer was basically a new instrument and we had only officially begun to operate it a few days before the discovery. So, maybe there were ways that such event candidates could be generated in the new detector itself or by something external. The main point is that we could measure the improved sensitivity but needed to run the detector for some length of time to determine whether events as observed could be some form of background. To test that required running for about one month and looking at off coincidence bins to see if such events are generated accidently. After doing this test for one month, we determined the probability of the event being an accidental was infinitesimal. This relieved one source of concern. The other was “how could we be fooled.” In this case, the worry we had was that maybe there was some way that someone had ‘injected’ a fake event into our data stream. We created a ‘tiger team’ of experts to investigate this, led by Matt Evans of MIT. They did a thorough job of tracing the signal back to each detector (meaning that it would have had to be generated and injected into the data at our two LIGO detector sites within 6.9 milliseconds of each other. They showed that this could not have happened in any way they could conceive.
At this point, everyone in LIGO, including me, decided the event was real and we should proceed toward publication, keeping the discovery totally internal until we published, so that we could present it as professionally as possible. We spent another month doing the physics analysis for fitting to general relativity, parameter estimation, etc. By early November, we began writing the discovery paper. It was completed in early December and Physical Review Letters said they could not do an expedited review with Christmas vacation coming so soon. So, we held the paper until January. In the interim, on December 26 we saw our second ‘five-sigma’ event. The black holes for this event were somewhat lighter and therefore the event went to higher frequencies and had many cycles in our frequency band. Any doubt that might have lingered in my colleague and my mind were set to rest with this observation.
For me, despite the fact that the first event made an incredibly strong case, I let out a final sigh of relief. This was not due to any lingering doubts about what we had done, but a history in physics of the difficulty in claiming a discovery based on one event. I recall the discovery of the W-based on a single event in a hydrogen bubble chamber beautifully established the quark model of particle physics. On the other hand, a claimed discovery of the magnetic monopole, again based on a single event, appeared convincing, but was not confirmed in much more sensitive experiments.
The announcement of our observation was made on February 11, 2016. The LIGO press conferences was in Washington DC and simultaneously I gave the first scientific seminar on our result at CERN. We were, of course, very pleased by the acceptance and enthusiasm for our discovery by the scientific community. That has continued through our observations of several more black hole mergers, and more recently, a binary neutron star merger. The neutron star merger event had electromagnetic counterparts. This combined science has been amazing and is the beginning of a new astronomy – multimessenger astronomy, which I believe will lead to exciting new understanding about our universe in the coming decades and beyond.
As I write this, we are working hard to make LIGO an even more sensitive instrument, as well as doing R&D and developing concepts for next generation gravitational wave detectors. This will keep me busy for the rest of my career, and I eagerly embrace my future!
This autobiography/biography was written at the time of the award and later published in the book series Les Prix Nobel/ Nobel Lectures/The Nobel Prizes. The information is sometimes updated with an addendum submitted by the Laureate.
Nobel Prizes and laureates
Six prizes were awarded for achievements that have conferred the greatest benefit to humankind. The 12 laureates' work and discoveries range from proteins' structures and machine learning to fighting for a world free of nuclear weapons.
See them all presented here.