Popular information
Popular science background:
Black holes and the Milky Way’s darkest secret (pdf)
Populärvetenskaplig information:
Om svarta hål och Vintergatans mörkaste hemlighet (pdf)
The Nobel Prize in Physics 2020
Three Laureates share this year’s Nobel Prize in Physics for their discoveries about one of the most exotic phenomena in the universe, the black hole. Roger Penrose showed that black holes are a direct consequence of the general theory of relativity. Reinhard Genzel and Andrea Ghez discovered that an invisible and extremely heavy object governs the stars’ orbits at the centre of our galaxy, the Milky Way. A supermassive black hole is the only currently known explanation.
Black holes and the Milky Way’s darkest secret
Roger Penrose invented ingenious mathematical methods to explore Albert Einstein’s general theory of relativity. He showed that the theory leads to the formation of black holes, those monsters in time and space that capture everything that enters them. Nothing, not even light, can escape.
Reinhard Genzel and Andrea Ghez each lead a group of astronomers who have focused on a region at the centre of the Milky Way since the early 1990s. With increasing precision, they have mapped the orbits of the brightest stars that are closest to the centre. Both groups found something that is both invisible and heavy, forcing this jumble of stars to swirl around. This invisible mass has about four million solar masses squeezed together in a region no larger than our solar system. What is it that makes the stars at the heart of the Milky Way swing around at such astonishing speeds? According to the current theory of gravity, there is only one candidate – a supermassive black hole.
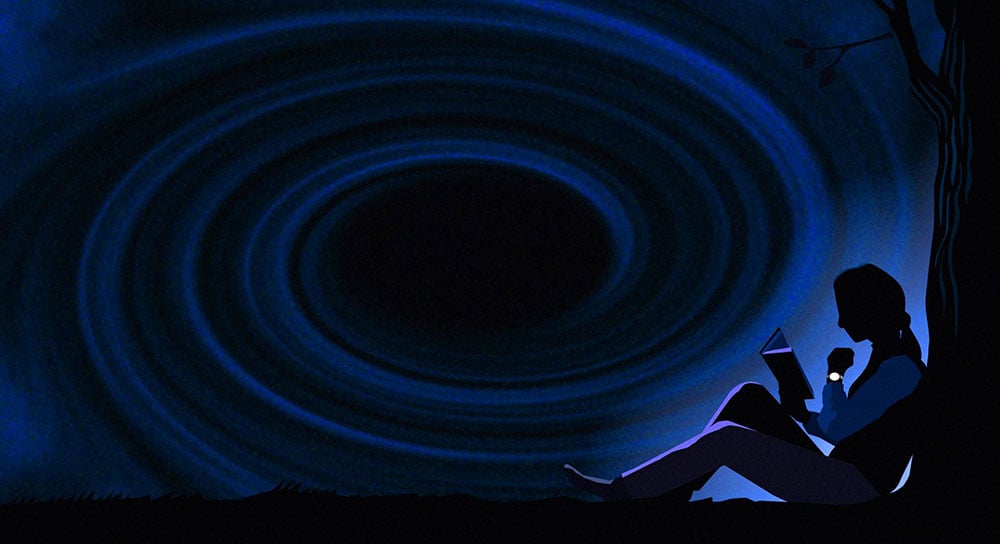
A breakthrough beyond Einstein
Not even Albert Einstein, the father of general relativity, thought that black holes could actually exist. However, ten years after Einstein’s death, the British theorist Roger Penrose demonstrated that black holes can form and described their properties. At their heart, black holes hide a singularity, a boundary at which all the known laws of nature break down.
To prove that black hole formation is a stable process, Penrose needed to expand the methods used to study the theory of relativity – tackling the theory’s problems with new mathematical concepts. Penrose’s ground-breaking article was published in January 1965 and is still regarded as the most important contribution to the general theory of relativity since Einstein.
Gravity holds the universe in its grip
Black holes are perhaps the strangest consequence of the general theory of relativity. When Albert Einstein presented his theory in November 1915, it upended all the previous concepts of space and time. The theory provided an entirely new foundation for understanding gravity, which shapes the universe at the largest scale. Since then, this theory has provided the basis for all studies of the universe, and also has a practical use in one of our most common navigation tools, the GPS.
Einstein’s theory describes how everything and everyone in the universe is held in the grip of gravitation. Gravity holds us on Earth, it governs the orbits of the planets around the Sun and the orbit of the Sun around the centre of the Milky Way. It leads to the birth of stars from interstellar clouds, and eventually their death in a gravitational collapse. Gravitation brings shape to space and influences the passage of time. A heavy mass bends space and slows time; an extremely heavy mass can even cut off and encapsulate a piece of space – forming a black hole.
The first theoretical description of what we now call a black hole came just a few weeks after the publication of the general theory of relativity. Despite the theory’s extremely complicated mathematical equations, the German astrophysicist Karl Schwarzschild was able to provide Einstein with a solution that described how heavy masses can bend space and time.
Later studies showed that once a black hole has formed, it is surrounded by an event horizon that sweeps around the mass at its centre like a veil. The black hole remains forever hidden inside its event horizon. The greater the mass, the larger the black hole and its horizon. For a mass equivalent to the Sun, the event horizon has a diameter of almost three kilometres and, for a mass like that of the Earth, its diameter is just nine millimetres.
A solution beyond perfection
The concept of the ‘black hole’ has found new meaning in many forms of cultural expression but, for physicists, black holes are the natural end point of the evolution of giant stars. The first calculation of the dramatic collapse of a massive star was made at the end of the 1930s, by physicist Robert Oppenheimer, who later led the Manhattan Project that constructed the first atomic bomb. When giant stars, many times heavier than the Sun, run out of fuel, they first explode as supernovas and then collapse into extremely densely packed remnants, so heavy that gravity pulls everything inside, even light.
The idea of ‘dark stars’ was considered as long ago as the end of the 18th century, in the works of the British philosopher and mathematician John Michell and the renowned French scientist Pierre Simon de Laplace. Both had reasoned that heavenly bodies could become so dense that they would be invisible – not even the speed of light would be fast enough to escape their gravity.
A little more than a century later, when Albert Einstein published his general theory of relativity, some of the solutions to the theory’s notoriously difficult equations described just such dark stars. Up until the 1960s, these solutions were regarded as purely theoretical speculations, describing ideal situations in which stars and their black holes were perfectly round and symmetrical. But nothing in the universe is perfect, and Roger Penrose was the first to successfully find a realistic solution for all collapsing matter, with its dints, dimples and natural imperfections.
The mystery of quasars
The question of the existence of black holes resurfaced in 1963, with the discovery of quasars, the brightest objects in the universe. For almost a decade, astronomers had been puzzled by radio rays from mysterious sources, such as 3C273 in the constellation of Virgo. The radiation in visible light finally revealed its true location – 3C273 is so far away that the rays travel towards Earth for over a billion years.
If the light source is such a long way away, it must have an intensity equal to the light of several hundred galaxies. It was given the name ‘quasar’. Astronomers soon found quasars that were so distant they had emitted their radiation in the early childhood of the universe. Where does this incredible radiation come from? There is only one way to obtain that much energy within the limited volume of a quasar – from matter falling into a massive black hole.
Trapped surfaces solved the riddle
Whether black holes could form under realistic conditions was a question that puzzled Roger Penrose. The answer, as he later recalled, appeared in the autumn of 1964 during a walk with a colleague in London, where Penrose was professor of mathematics at Birkbeck College. When they stopped talking for a moment to cross a side street, an idea flashed into his mind. Later that afternoon, he searched for it in his memory. This idea, which he called trapped surfaces, was the key he had unconsciously been searching for, a crucial mathematical tool needed to describe a black hole.
A trapped surface forces all rays to point towards a centre, regardless of whether the surface curves outwards or inwards. Using trapped surfaces, Penrose was able to prove that a black hole always hides a singularity, a boundary where time and space end. Its density is infinite and, as yet, there is no theory for how to approach this strangest phenomenon in physics.
Trapped surfaces became a central concept in the completion of Penrose’s proof of the singularity theorem. The topological methods he introduced are now invaluable in the study of our curved universe.
A one-way street to the end of time
Once matter begins to collapse and a trapped surface forms, nothing can stop the collapse from continuing. There is no way back, as in the story told by physicist and Nobel Laureate Subrahmanyan Chandrasekhar, from his childhood in India. The story is about dragonflies and their larva, which live underwater. When a larva is ready to unfold its wings, it promises it will tell its friends what life is like on the other side of the water’s surface. But once the larva passes through the surface and flies away as a dragonfly, there is no return. The larvae in the water will never hear the story of life on the other side.
Similarly, all matter can only cross a black hole’s event horizon in one direction. Time then replaces space and all possible paths point inwards, the flow of time carrying everything towards an inescapable end at the singularity (figure 2). You will not feel anything if you fall through the event horizon of a supermassive black hole. From the outside, no one can see you falling in and your journey towards the horizon continues forever. Peering into a black hole is not possible within the laws of physics; black holes hide all their secrets behind their event horizons.
Black holes govern the paths of stars
Even though we cannot see the black hole, it is possible to establish its properties by observing how its colossal gravity directs the motions of the surrounding stars.
Reinhard Genzel and Andrea Ghez each lead separate research groups that explore the centre of our galaxy, the Milky Way. Shaped like a flat disc about 100,000 light years across, it consists of gas and dust and a few hundred billion stars; one of these stars is our Sun (figure 3). From our vantage point on Earth, enormous clouds of interstellar gas and dust obscure most of the visible light coming from the centre of the galaxy. Infrared telescopes and radio technology were what first allowed astronomers to see through the galaxy’s disc and image the stars at the centre.
Using the orbits of the stars as guides, Genzel and Ghez have produced the most convincing evidence yet that there is an invisible supermassive object hiding there. A black hole is the only possible explanation.
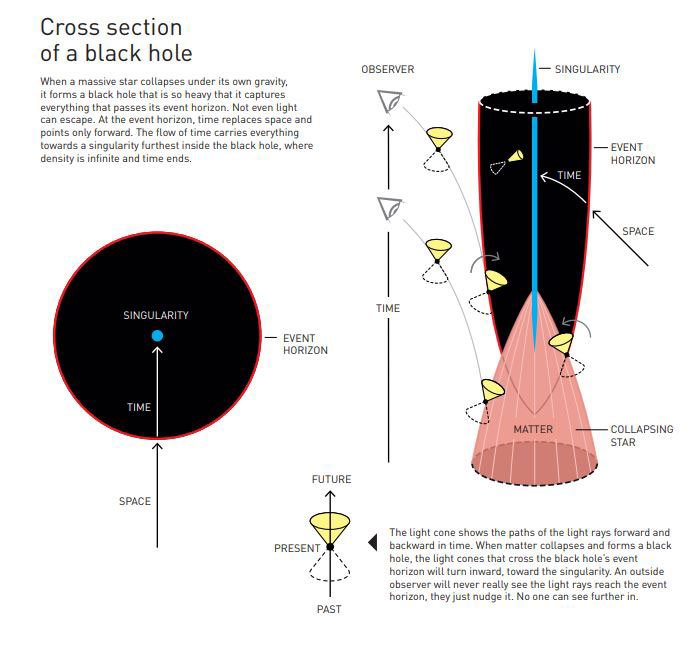
Figure 2. A black hole is formed.
Focus on the centre
For more than fifty years, physicists have suspected that there may be a black hole at the centre of the Milky Way. Ever since quasars were discovered in the early 1960s, physicists reasoned that supermassive black holes might be found inside most large galaxies, including the Milky Way. However, no one can currently explain how the galaxies and their black holes, between a few million and many billion solar masses, were formed.
One hundred years ago, the American astronomer Harlow Shapley was the first to identify the centre of the Milky Way, in the direction of the constellation of Sagittarius. With later observations astronomers found a strong source of radio waves there, which was given the name Sagittarius A*. Towards the end of the 1960s, it became clear that Sagittarius A* occupies the centre of the Milky Way, around which all stars in the galaxy orbit.
It was not until the 1990s that bigger telescopes and better equipment allowed more systematic studies of Sagittarius A*. Reinhard Genzel and Andrea Ghez each started projects to attempt to see through the dust clouds to the heart of the Milky Way. Along with their research groups, they developed and refined their techniques, building unique instruments and committing themselves to long-term research.
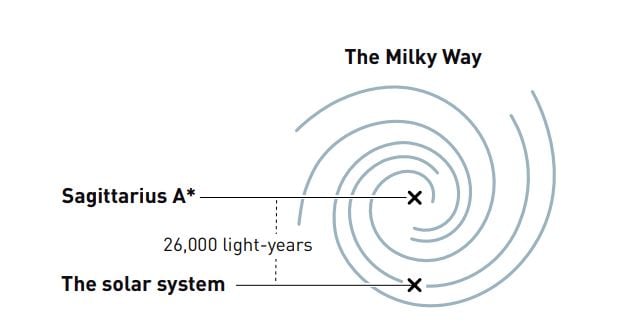
Figure 3. The Milky Way, our galaxy, seen from above. It is shaped like a flat disc about 100,000 light-years across. Its spiral arms are made of gas and dust and a few hundred billion stars. One of these stars is our Sun.
Only the world’s biggest telescopes will suffice for gazing at distant stars – the bigger the better is absolutely true in astronomy. The German astronomer Reinhard Genzel and his group initially used NTT, the New Technology Telescope on La Silla mountain in Chile. They eventually moved their observations to the Very Large Telescope facility, VLT, on Paranal mountain (also in Chile). With four giant telescopes twice the size of NTT, the VLT has the world’s biggest monolithic mirrors, each with a diameter of more than 8 metres.
In the USA, Andrea Ghez and her research team use the Keck Observatory, located on the Hawaiian mountain of Mauna Kea. Its mirrors are almost 10 metres in diameter and are currently among the largest in the world. Each mirror is like a honeycomb, consisting of 36 hexagonal segments that can be controlled separately to better focus the starlight.
The stars show the way
However big the telescopes, there is always a limit to the detail they can resolve because we live at the bottom of an almost 100-kilometre-deep atmospheric sea. Large bubbles of air above the telescope, which are hotter or colder than their surroundings, act like lenses and refract the light on its way to the telescope’s mirror, distorting the light waves. This is why the stars twinkle and also why their images are blurred.
The advent of adaptive optics was crucial in improving observations. The telescopes are now equipped with a thin extra mirror that compensates for the air’s turbulence and corrects the distorted image.
For almost thirty years, Reinhard Genzel and Andrea Ghez have followed their stars in the distant stellar jumble at the centre of our galaxy. They continue to develop and refine the technology, with more sensitive digital light sensors and better adaptive optics, so that image resolution has improved more than a thousandfold. They are now able to more precisely determine the stars’ positions, following them night by night.
The researchers track some thirty of the brightest stars in the multitude. The stars move most rapidly within a radius of one light-month from the centre, inside which they perform a busy dance like that of a swarm of bees. The stars that are outside this area, on the other hand, follow their elliptical orbits in a more orderly manner (figure 4).
One star, called S2 or S-O2, completes an orbit of the centre of the galaxy in less than 16 years. This is an extremely short time, so the astronomers were able to map its entire orbit. We can compare this to the Sun, which takes more than 200 million years to complete one lap around the Milky Way’s centre; dinosaurs were walking the Earth when we started our current lap.
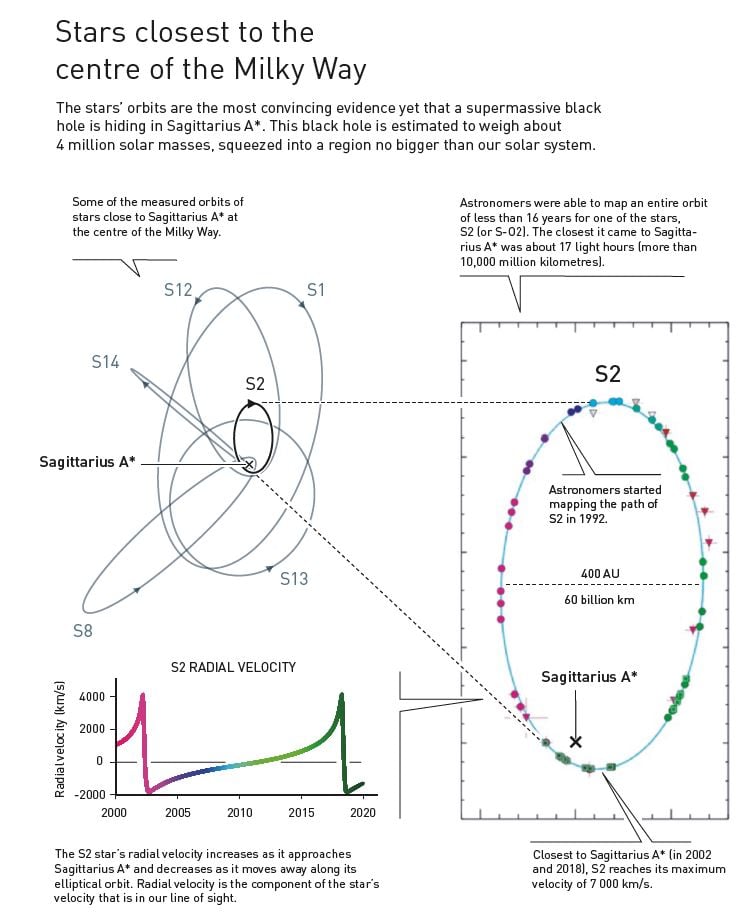
Figure 4. The stars’ orbits revealed that something invisible and heavy governed their paths at the heart of the Milky Way.
Theory and observations follow each other
The agreement between the measurements of the two teams was excellent, leading to the conclusion that the black hole at the centre of our galaxy should be equivalent to around 4 million solar masses, packed into a region the size of our solar system.
We may soon get a direct look at Sagittarius A*. This is next on the list because, just over a year ago, the Event Horizon Telescope astronomy network succeeded in imaging the closest surroundings of a supermassive black hole. Farthest in, in the galaxy known as Messier 87 (M87), 55 million light years from us, is a blacker than black eye surrounded by a ring of fire.
The black core of M87 is gigantic, more than one thousand times heavier than Sagittarius A*. The colliding black holes that caused the recently discovered gravitational waves were considerably lighter. Like black holes, gravitational waves existed only as calculations from Einstein’s general theory of relativity, before being captured for the first time in the autumn of 2015, by the LIGO detector in the USA (Nobel Prize in Physics, 2017).
What we do not know
Roger Penrose showed that black holes are a direct consequence of the general theory of relativity but, in the infinitely strong gravity of the singularity, this theory ceases to apply. Intensive work is being conducted in the field of theoretical physics to create a new theory of quantum gravity. This must unite the two pillars of physics, the theory of relativity and quantum mechanics, which meet in the extreme interior of black holes.
At the same time, observations are coming closer to black holes. The pioneering work of Reinhard Genzel and Andrea Ghez has led the way for new generations of precise tests of the general theory of relativity and its most bizarre predictions. Most likely, these measurements will also be able to provide clues for new theoretical insights. The universe has many secrets and surprises left to be discovered.
Further reading
Additional information on this year’s prizes, including a scientific background in English, is available on the website of the Royal Swedish Academy of Sciences, www.kva.se, and at www.nobelprize.org, where you can watch video footage of the press conferences, the Nobel Lectures and more. Information on exhibitions and activities related to the Nobel Prizes and the Prize in Economic Sciences is available at www.nobelprizemuseum.se
The Royal Swedish Academy of Sciences has decided to award the Nobel Prize in Physics 2020
with one half to
ROGER PENROSE
Born 1931 in Colchester, UK. Ph.D.
1957 at University of Cambridge, UK.
Professor at University of Oxford, UK
“for the discovery that black hole formation is a robust prediction of the general theory of relativity”
and the other half jointly to
REINHARD GENZEL
Born 1952 in Bad Homburg vor
der Höhe, Germany. Ph.D. 1978
at University of Bonn, Germany.
Director at Max Planck Institute for
Extraterrestrial Physics, Garching,
Germany and Professor at University
of California, Berkeley, USA.
and
ANDREA GHEZ
Born 1965 in City of New York, USA.
Ph.D. 1992 at California Institute of
Technology, Pasadena, USA.
Professor at University of California,
Los Angeles, USA.
“for the discovery of a supermassive compact object at the centre of our galaxy”
Science Editors: Ulf Danielsson, Ariel Goobar, David Haviland and Gunnar Ingelman, the Nobel Committee for Physics
Text: Joanna Rose
Translator: Clare Barnes
Illustrations: ©Johan Jarnestad/The Royal Swedish Academy of Sciences
Editor: Sara Gustavsson
© The Royal Swedish Academy of Sciences
Nobel Prizes and laureates
Six prizes were awarded for achievements that have conferred the greatest benefit to humankind. The 12 laureates' work and discoveries range from proteins' structures and machine learning to fighting for a world free of nuclear weapons.
See them all presented here.